The New Biology
THE NEW BIOLOGY
Michael Levin,PHD Tufts University.
Non-neural Bioelectricity Techniques to Manipulate the Layer of Physiological Software
Your brain your body tissues form electrical networks that make decisions these are decisions about dynamic anatomy and that we now have the ability to target the system to control large-scale editing that can even override all kinds of genomic default states with lots of advantages and opportunities for regenerative medicine and synthetic bioengineering
we all start life roughly like:
A collection of embryonic blastomeres and then thereafter a complex process----- you get something like a cross section through a human torso- think about this incredible invariant order all of these organs are the correct shape size -position - orientation everything is next to the right stuff. It's absolutely staggering amount of amount of order
We know that genomes specify the DNA specifies proteins there's nothing directly that specifies how is it that cells use the genomically specified hardware that they have to know what to make and when to stop. in regenerative medicine we would like to know if a piece of this is missing how do we get the cells to rebuild it
A single cell organism called the lacrimaria HAS is no brain -there's no nervous system t- no stem cells there's no cell to cell communication-- just one cell handling all of its local goals
Their ability to pursue states in various kinds of problem spaces, including anatomical amorphous space, physiological space BUT a single cell can give rise to a collection of cells -that self-assembles into incredibly complex morphology.
In a standard developmental biology ,that we're told is a kind of feed-forward ,open-loop system which is very much based around "emergence".
When a singular cell is operating at this level ,we must ask about the algorithms-- how are these decisions being made what do the cellular collectives measure, what are , are there modules ,or subroutines ,how are these global patterning goals specified ,and stored and,how reprogrammable is any piece of the biological hardware involved
This information comes in many flavors so chemical extracellular matrix tensions and stresses or biomechanics all kinds of things and of course bioelectricity so bioelectricity doesn't do this alone it works with all of this other stuff
It's actually a computational layer that gives some privileged access to the control of complex anatomical features it is the medium in which the computations are being made to make decisions
You have a collection of cells ,each cell has ion channels in this in the plasma membrane they set voltage values across- that's produces the resting potential ,which can go up and down, and can it propagate to the neighbors via these electrical synapses. Those kind of networks underlie this amazing software ,
We need to understand the electrical activity, the commitment of neuroscience , if we are able to decode this .
Functional cognitive structures are to be read out from the electrical activity of the brain that's what we believe well it turns out that actually all cells do this okay all cells have ion channels most cells have electrical synapses known as gap junctions to their neighbors and we have undertook uh undertaken this this project to try to do the same kind of decoding so
an embryo and we would like to read all the electrical conversations that bind the individual cells into a collective that uh can undertake undergo anatomical homeostasis towards large uh states like like build a limb build a kidney and and so on and on the one hand it'
strange and surprising to many people to think about somatic cells as having this kind of neuroscience-like um aspect to them as if they're processing information they're having having goal states
we know what ion channels and pumps are in the membrane can we explain why the voltage is the way it is and why it changes as a function of time the way it does and so let me show you a couple of native bioelectrical patterns
the bioelectrical properties set up a pre-pattern a scaffold that tell you where everything is going to be
once you're electrically disconnected from this grid the rest of the animal becomes just external
you can control in any tissue you can control which cells electrically couple to which other cells and so we target these gap junctions we can mutate them we can open them close them or and this is basically synaptic the controlling synaptic plasticity or we can control the various ion channels opening again opening and closing them to actually set the voltage of the individual cells and this would be the equivalent of some sort of intrinsic plasticity
these bioelectrical states are not just readouts just yet molecular machinery but the information-bearing medium for these large-scale modifications so one thing you can do is you can take some cells in the frog that are going to become gut
in this region that's going to be part of the gut we'll set up a voltage pattern a distribution of voltage that's very similar to what happens when the native eyes if you recapitulate that pattern somewhere else the cells are using that pattern to decide what they're going to build and that pattern tells them to build an eye and here they go they will build an eye out of endodermal cells e round lens so so it's a non-cell autonomous effect and when we see this again and again that there's this this this ability of these bioelectrical signals to uh not just to specify cell fate but but to actually specify organ type and position and things like that so we can make eyes we can make h what this is all about is is predictive control over large-scale anatomy the idea is that we can show how much of this is actually under bioelectrical control and then use computational techniques to gain uh to gain good good predictive control over
this tissue already knows how to do and the decision for what it's going to do scar or produce various types of organs can be is is part of an electric circuit that we can guide we can we can we can manage
the second thing is that in keeping with this idea from the beginning that that one of the hallmarks of the collective intelligence of these cells in in responding to novel situations is that they can get to the final outcome through through novel paths and if you actually look at this at this intermediate stage of this leg this is nothing like what frog
we've done we've done some work um with david kaplan and um lauren black and others uh on human mesenchymal stem cells and cardiomyocytes and you can control the kinds of things that you can see in vitro um differentiation proliferation wound healing but i think that's not what the bioelectricity is for what the bioelectricity is really for in terms of why evolution uses
we should be interested in is because it allows us to exploit the modular nature of the anatomical decision making because we can control very large scale outcomes not micromanage the the um the details and uh here i have to do a disclosure so david kaplan and i are both um co-founders of this company called morphaceuticals inc where we're trying to take the things that we learned about frog leg regeneration and move them towards mammals so our goal is net where we're now in rodents trying for limb regeneration using the same strategies and using these wearable bioreactors
it is already known how it is that voltage change impacts the transcriptional machinery --at if we understood how voltage controls gene expression we basically do there are we already know about six ways that that voltage change transduces down into second messenger pathways and controls gene expression
The voltage-sensitive phosphatases neurotransmitter transporters we already know how this works at a single cell level this transduction machinery -all of these kinds of things are in fact the redistribution of morphogens in the examples that i showed you uh all of this this is known both from specific candidates and
what allows us to do is to build models of both molecular uh networks like grns together with the various biophysics steps that happen and you can simulate every cell having these various circuits and then you can try to build a full stack model that integrates from the molecular steps to the tissue level physiology like every cell has various channels what is the electrical pattern and going to be in this tissue
i think is the kind of the the future of using this for regenerative medicine
the cancer issue so so i've shown you that you can you can track the uh the the the the shrinking of these kinds of um computational boundaries from from from that of the organ or whole body scale to single cells and you can use this as there's an obvious uh application here as a kind of diagnostic modality right um is screening for cancer and so on
but functionally what's what's really important is that first of all you can induce basically a a conversion of normal melanocytes
instructor cells for an obvious reason they those cells tell the melanocytes what to do in particular to stay nice cooperative melanocytes under control if you block their ability to signal what happens is basically metastatic melanoma these normal melanocytes convert to these crazy uh really really um kind of a long stringy things that start to crawl a crawl away they dig into the brain they dig into the neural tube they start to invade here they are all through the blood vessels this is basically exactly like a melanoma type of behavior
and the melanocytes are just at this point they're they're they're going on their own so it's a voltage change in the environment --there's nothing genetically wrong with these animals there are no oncogenes there were no carcinogens no um uh nothing nothing like that but but this voltage change is this is a physiological switch away from the cooperation of cells toward embryogenesis and towards single cell
despite what the oncogene is trying to get them to do and here while while that uh dominant negative uh k ras p53 whatever while they're uh blazingly expressed there's no tumor it's the same animal there's no tumor because these cells are coupled to this electrical network that forces them towards a uh to a proper tissue
uh try to use this to target target carcinogenic behavior in human cells the other thing that is important is again to think about the the level of control that's possible with this
you can build a computational model that asks a simple question what determines the shape and size of the brain and there's a particular bioelectrical pattern that's required for the brain to get back to the correct you can get back to a normal brain shape a normal brain gene expression
choose your tissue and and so on and the idea is that something like 20 of all drugs are ion channel drugs and these things form an incredibly convenient toolkit of what we call them electroceuticals because if you have the right computational model you can repurpose these they have a massive applicability in tweaking the bioelectrical signals now another nice thing about this kind of approach is that once the bioelectric circuit has made its decision the downstream steps that are in second messengers than gene expression everything else it can be as long as you want
we think that cracking this bioelectric codes can help reveal how cell networks make decisions in large-scale anatomy not just how individual cells decide what type of cell they're going to be but actually the question of growth and form where where do these comple patterns reside ,and we can now rewrite some of these patterns for all kinds of uh all kinds of applications ollaborators
Michael Levin References
https://en.wikipedia.org/wiki/Michael_Levin_(biologist)
https://www.youtube.com/watch?v=p3lsYlod5OU&t=3932s
https://www.youtube.com/watch?v=WM8bQWfmeB8
https://www.youtube.com/watch?v=XheAMrS8Q1c&t=257s
https://www.youtube.com/watch?v=NJFMbLrazXw&t=11s
https://www.youtube.com/watch?v=KfrEDUv29r0
https://www.youtube.com/watch?v=AC2_S-wcJes&t=36s
https://www.youtube.com/watch?v=ZSddchIGNG0&t=5s
https://www.youtube.com/watch?v=XHMyKOpiYjk&t=19s
https://www.youtube.com/watch?v=BZCSExf4ZWc&t=7s
https://www.youtube.com/watch?v=Y3VI643ZtZY
https://www.youtube.com/watch?v=XheAMrS8Q1c
https://www.youtube.com/watch?v=V89_8-H6IIM
https://www.youtube.com/watch?v=JC4FOzAuHF4&t=33s
https://www.youtube.com/watch?v=JC4FOzAuHF4
https://www.youtube.com/watch?v=V89_8-H6IIM
https://www.youtube.com/watch?v=XHMyKOpiYjk
https://www.youtube.com/watch?v=AC2_S-wcJes&t=116s
https://www.youtube.com/watch?v=AC2_S-wcJes&t=116s
https://www.youtube.com/watch?v=xsy8p435DqM&t=5195s
https://www.youtube.com/watch?v=KfrEDUv29r0&t=35s
https://www.youtube.com/watch?v=Ed3ioGO7g10&t=1360s
https://www.youtube.com/watch?v=_QICRPFWDpg&t=1673s
https://www.youtube.com/watch?v=5XvDdbYzwf8
For this webpage I reviewed many up-to-date scientific papers by certified and academically sound scientists – some Nobel prize winners.
The most mind moving presentations I reviewed were by Michael Levin.
He is primarily interested in tissue – limb regeneration (i.e. how a salamander or lizard regenerates their cut off tales).
He discovered a natural bioelectrical phenomenon that assisted this project and since that time introduce a whole concept of bioelectric patterns and biology and possibly medicine
In the past we considered that individual cells must have some kind of communication with each other ,but felt it was more on an interactive chemistry basis (i.e. Turing – self organization, Assembly theory) but Levin and his group has discovered that preformed “electrical matrix patterns” do exist in nature. These patterns when imprinted on tissue or cells can form, or reform, organs in a pre-prescribed manner as shown in his laboratory studies. In many conversations Levin has with others scientists, the question that comes up is --where does this preformed pattern “come from”. Levin proposes that these patterns exist in nature , conceptually ,as if it were a " Plato- like preformed abstract." Levin is currently clinically applying his techniques to manage the patterns for tissue regeneration, and possibly the treatment of cancer by reforming cell structure.It also,in doing reveals another force in our natural lives that we have had little awareness of ----------
EXTENSIVE LIST OF UTUBE LECTURES AT END OF THIS PAGE
People tend to think of intelligence as being of two kinds: There's the quote, unquote "real" intelligence, which is what we are supposed to have, and then there's this idea of a 'collective intelligence,' so swarms of bees, colonies of ants, shoals of fish, and so on. And people tend to think of those as radically different things. But the reality is all intelligence is collective intelligence, and this is because we are all made of parts. So you and I are collections of cells, and these cells, including neurons and various other cells in our body, have many competencies- this is because they were once separate individuals by themselves.
They were unicellular organisms with all of the skills needed to survive in a complex world. And that journey that we all took, those progressive steps by which we construct ourselves- we construct our bodies, we construct our minds- that journey is maybe the most profound question in all of science.
I'm Michael Levin, and I'm a developmental biologist at Tufts University. Developmental biology is maybe the most magical of all the sciences because you get to see with your own eyes that journey that we all take from physics to mind. We all start out life as an unfertilized oocyte, and then slowly, gradually, step-by-step, that oocyte turns into a bunch of cells that self-construct an embryo, and eventually that embryo matures and becomes a large-scale adult. In the case of a human, it will be an individual with metacognitive capacities and the ability to reason. But we all have our origin in that chemistry and physics of that initial oocyte.
And the magic of developmental biology is that there is a mechanism by which all of these cells get together, and they are able to cooperate towards large-scale goals. This is the notion that biology uses what I call a "Multi-scale competency architecture," which basically means that we are not simply nested structurally in terms of cells which comprise tissues, comprising organs, and bodies, and then ultimately societies and so on- that's obviously true on a structural level.
But more interesting is the fact that each of these layers has certain problem-solving competencies. Each one solves problems in their own space, so cells are simultaneously solving problems in physiological spaces and metabolic spaces and gene expression spaces, and tissues and organs are solving those problems.
But, for example, during embryogenesis or regeneration, they're also solving problems in anatomical space. They're trying to navigate a path from the shape of an early embryo or a fertilized zygote all the way up to the complexity of a human body with all of the different types of organs and structures
So the competency architecture refers to the fact that all of the parts inside of us and inside of all other creatures are themselves competent agents with preferences, with goals, with various abilities to pursue those goals, and other types of problem-solving capacities.
What evolution has given us is this remarkable architecture where every level shapes the behavioral landscape of the levels below- and the levels below do clever and interesting things that allow the levels above not to have to micromanage, and to be able to control in an interesting top-down capacity. One of the most important things about this emerging field of diverse intelligence is that we, as humans, have very limited capacity and finely-honed ability to see intelligence in medium-sized objects moving at medium speeds through three-dimensional space. So we see other primates and we see crows and we see dolphins, and we have some ability to recognize intelligence.
But we really are very bad at recognizing intelligence in unconventional embodiments where our basic expectations strain against this idea that there could be intelligence in something extremely small or extremely large.
People often criticize this approach by saying, "Well, then anything goes. If you can pick up a rock and say, 'I think this rock is cognitive and intelligent, you know, there's a spirit with hopes and dreams inside of every rock.'" That's not what this is. This is quite different. As an engineer, what you have to do is you have to come up with a way to look at a particular system that doesn't overestimate its intelligence or underestimate its intelligence. If you treat complex animals as if they were clockwork, you're gonna miss everything that's important and exciting about how they work. If you treat a clock as if it had a complex intelligence, you're going to waste a lot of time- but getting it right is fundamentally important. And so, you have this spectrum where the engineer has to pick the right level for the right system, and it's critical not to then say, "Well, that doesn't sound like human intelligence," right?
We're looking for the basic minimal version. So I think it should have two things: The first thing it should have is some degree of goal-directedness, some ability to take different paths to get to the same goals; so this is William James's definition of intelligence. And it has to have some ability to undertake actions that are not completely determined by local circumstances.
So you start with all of that, and systems that have scaled up, those basic, very fundamental, non-zero levels of agency, we call that life. So it is a spectrum, and I think that in this Universe it goes all the way to the bottom. It's very hard for people to think about these unconventional kinds of intelligences that may be either too large or too small. And of course, they often work in other spaces.
So we're good with three-dimensional space, but imagine if we had a primary sense of our own blood chemistry. If you could feel your blood chemistry the way that you currently see and smell and taste things that are around you, I think we would have absolutely no problem having an intuitive understanding of physiological-state space the way we do for three-dimensional space.
I think that we would immediately be able to recognize our various internal organs as intelligent agents navigating that space and solving these kind of problems, all the various things that happen during the day. And here's one of my favorite examples of problem solving in physiological and transcriptional spaces: So we have these worms. These are Planaria, these are flatworms. And if you put these flatworms in a solution of barium- barium is a non-specific potassium channel blocker; it blocks the ability of these cells to exchange potassium with the outside world- the cells really don't like it, especially cells in the head, because the head is full of neurons; neurons love to pass potassium.
And so what happens is that overnight their heads explode. Literally, it's called head deprogression; they literally lose their heads. But if you leave them in the barium, within a couple weeks they grow back a new head. And the new head is completely barium-adapted, has no problem with the barium whatsoever. We said, "How could this be?" We looked at the original heads, the original naive heads, we looked at the barium-adapted heads, and we simply did a subtraction and asked, "What are the different genes that are expressed here?" And we found out that the barium-adapted heads have just a small number of genes that were turned on and off to allow them to adapt to this novel stressor.
Now, here's the amazing part. Planaria never see barium in the wild. There's no evolutionary history of knowing what to do when you're hit with barium. This is not an ecological stressor that they normally have to deal with. So just imagine you're a bunch of cells and you're hit with this incredible physiological stressor you've never seen before.
You've got tens of thousands of genes. What do you do? How do you know which genes to turn on and off? You don't have time to try combinations. You don't have time for an exhaustive search. You don't have time for hill climbing. You don't have time to try random things because you'll probably kill the cell long before you solve the problem.
And yet, you are able to navigate from where you are to where you need to be to escape this physiological stressor. And so, what I think evolution has done is pivoted some of the same tricks from very simple systems that only solve metabolic problems eventually to physiological and then to transcriptional problems.
And when multicellularity comes on the scene, large-scale anatomical problems. And so it's never a question of: Is something physics and chemistry, or is it cognitive? The question is: What kind of cognition, and how much?
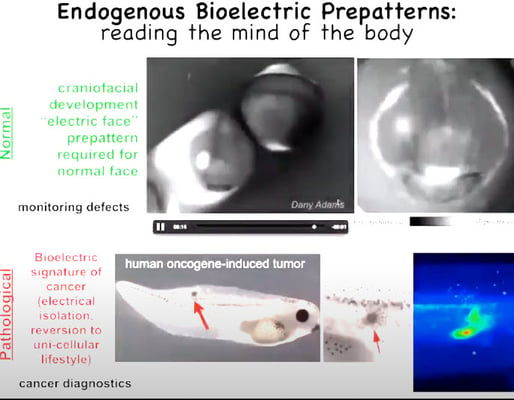
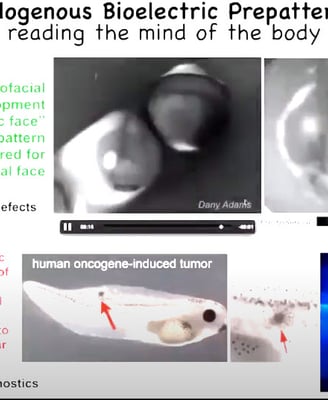
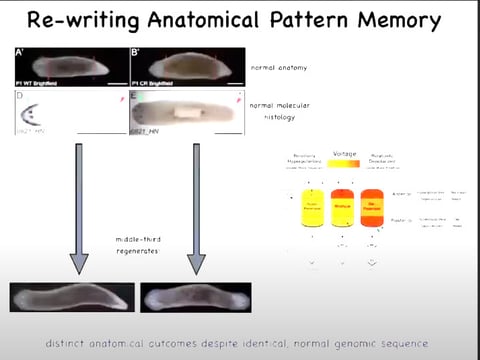
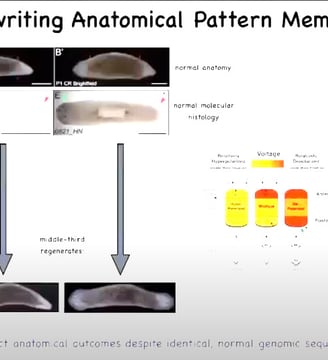
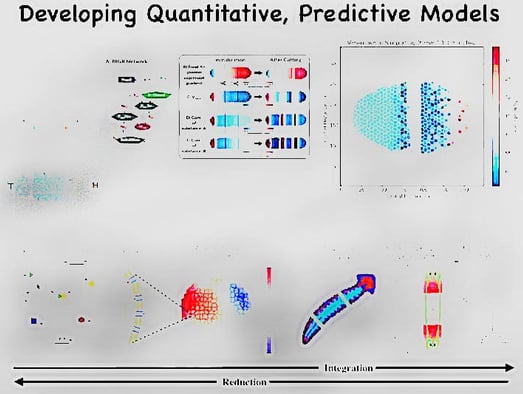
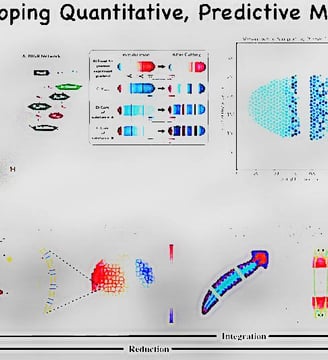
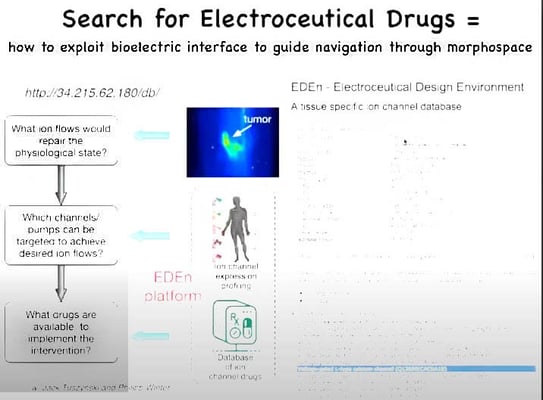
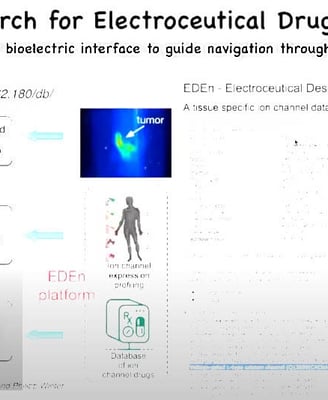