NEUROTRANSMITTERS
THE HUMAN BRAIN IS AWASH WITH NEUROTRANSMITTERS AND NEUROMODULATORS
WHILE AWAKE- THEY PLAY IMORTANT ROLES IN REGULATING BEHAVIOR AND EMOTIONS IN ALL HUMANS,
REGARDLESS OF THEIR RACE OR CULTURAL BACKGROUND.
The human brain is a highly complex organ, and behavior and emotions are influenced by a wide range of neurochemical Substances, many of which are not specific to any particular group of people.
However, it's important to note that the influence of neurochemicals on behavior and emotions is a complex interplay of various factors, including genetics, environment, and individual differencess
important to note that behaviors like aggression, acquisition, and survival instincts are complex and multifaceted, and they are not solely determined by a single neurotrophin or neurotransmitter.
Instead, they result from the interaction of multiple neural systems, genetic factors, environmental influences, and individual differences. The brain's regulation of these behaviors is a complex process involving various regions, circuits, and chemical messengers working together.Research in this area is ongoing, and our understanding of the neural mechanisms underlying these behaviors continues to evolve.
It's important to emphasize that the role of these neurochemicals in behavior and emotions is highly interconnected and influenced by a multitude of factors. Additionally, there may be cultural and genetic variations in how these neurotransmitters function or how they are regulated, but the fundamental neurochemical processes are shared among all humans. The idea of "indigenous neurotrophic substances" invoking behavior and emotions is not a well-established concept in mainstream neuroscience.
The neurotrophins, which include nerve growth factor (NGF), brain-derived neurotrophic factor (BDNF), and others, primarily play roles in the growth, development, and survival of neurons.
They are not typically associated with directly regulating behaviors like aggression, acquisition, or long-term survival instincts. Instead, these behaviors are influenced by a complex interplay of various neurotransmitters, hormones, and brain regions.
That being said, several neurotransmitters and neuromodulators have been implicated in regulating AGGRESSION AND SURVIVAL INSTINCTS
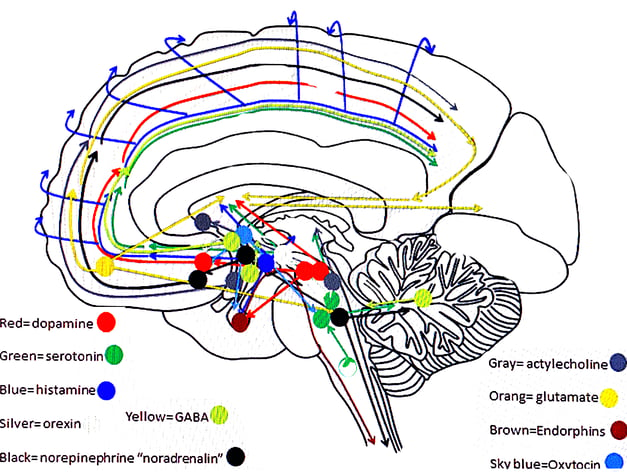
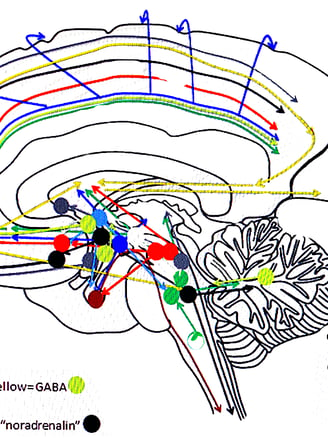
TRANSMITTER PATHWAYS FROM MIDBRAIN
ACTIVATION AT SYNAPSE (TARGET NEURON)
NEURO TRANSMITTERS -GENERAL
CLICK IMAGE
Introduction to Neurotransmitters Endogenus substances that invoke behavior and emotions in the human brain
The human brain is a highly complex organ, and behavior and emotions are influenced by a wide range of neurochemical substances, many of which are not specific to any particular group of people. However, it's important to note that the influence of neurochemicals on behavior and emotions is a complex interplay of various factors, including genetics, environment, and individual differences.
That being said, some neurotransmitters and neuromodulators play important roles in regulating behavior and emotions in all humans, regardless of their indigenous or cultural background. Some of these include:
1. Serotonin: Serotonin is often associated with mood regulation. Imbalances in serotonin levels have been linked to mood disorders like depression and anxiety. Medications like SSRIs (Selective Serotonin Reuptake Inhibitors) are commonly used to modulate serotonin levels.
2. Dopamine: Dopamine is involved in the brain's reward system and is associated with feelings of pleasure and motivation. Dysregulation of dopamine has been implicated in conditions such as addiction, schizophrenia, and certain mood disorders.
3. Norepinephrine (noradrenaline): Norepinephrine is involved in the "fight or flight" response and plays a role in arousal, attention, and alertness. It can influence mood and emotional responses.
4. GABA (Gamma-Aminobutyric Acid): GABA is an inhibitory neurotransmitter that helps regulate anxiety and stress by reducing neuronal excitability. Drugs that enhance GABA's effects are sometimes used to treat anxiety disorders.
5. Glutamate: Glutamate is the primary excitatory neurotransmitter in the brain and is involved in many aspects of brain function, including learning and memory. Dysregulation of glutamate can be associated with conditions like epilepsy and certain mood disorders.
6. Endorphins: Endorphins are endogenous opioids that are released during exercise, excitement, and stress. They play a role in pain modulation and can induce feelings of euphoria.
7. Oxytocin: Oxytocin is often referred to as the "love hormone" or "bonding hormone" because it plays a role in social bonding, attachment, and trust. It can influence emotional responses in social contexts.
It's important to emphasize that the role of these neurochemicals in behavior and emotions is highly interconnected and influenced by a multitude of factors. Additionally, there may be cultural and genetic variations in how these neurotransmitters function or how they are regulated, but the fundamental neurochemical processes are shared among all humans. The idea of "indigenous neurotrophic substances" invoking behavior and emotions is not a well-established concept in mainstream neuroscience.
The Neurotrophins that are the most involved with aggression , acquisition, and long term survival instincts
The neurotrophins, which include nerve growth factor (NGF), brain-derived neurotrophic factor (BDNF), and others, primarily play roles in the growth, development, and survival of neurons. They are not typically associated with directly regulating behaviors like aggression, acquisition, or long-term survival instincts. Instead, these behaviors are influenced by a complex interplay of various neurotransmitters, hormones, and brain regions.
That being said, several neurotransmitters and neuromodulators have been implicated in regulating behaviors such as aggression and survival instincts:
1. Serotonin: Serotonin is often associated with mood regulation, and imbalances in serotonin levels can influence aggression. Low serotonin levels have been linked to increased impulsivity and aggressive behaviors in some studies.
2. Dopamine: Dopamine is involved in the brain's reward system and can play a role in motivation and aggression. Abnormal dopamine levels or function have been associated with aggression in some cases.
3. Testosterone: Testosterone is a hormone that can influence aggression, particularly in males. Higher levels of testosterone have been linked to increased aggressive behaviors in some studies.
4. Cortisol: Cortisol is a stress hormone that can affect various aspects of behavior and physiology. Elevated cortisol levels in response to stress may influence survival instincts and decision-making.
5. Oxytocin and Vasopressin: These neuropeptides have been implicated in social behaviors, including aggression. Oxytocin is often associated with prosocial behaviors, while vasopressin may play a role in aggressive and territorial behaviors.
6. Norepinephrine (noradrenaline): Norepinephrine can be involved in the "fight or flight" response and may influence aggressive behaviors in certain situations.
It's important to note that behaviors like aggression, acquisition, and survival instincts are complex and multifaceted, and they are not solely determined by a single neurotrophin or neurotransmitter. Instead, they result from the interaction of multiple neural systems, genetic factors, environmental influences, and individual differences. The brain's regulation of these behaviors is a complex process involving various regions, circuits, and chemical messengers working together.
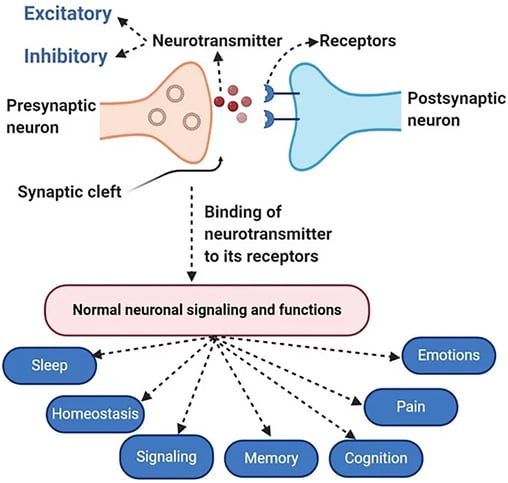
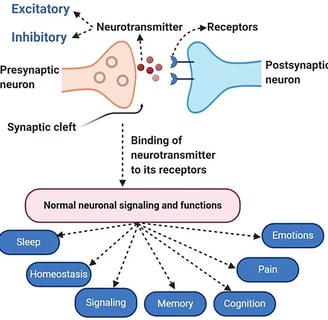
Dopamine is a neurotransmitter that plays a significant role in influencing human emotions and behavior. Its impact on emotions and behavior is complex and can vary depending on the context, the specific brain regions involved, and individual differences. Here are some key ways in which dopamine affects human emotions and behavior:Dopamine is involved in the brain's reward system and is associated with feelings of pleasure and motivation. Dysregulation of dopamine has been implicated in conditions such as addiction, schizophrenia, and iIT an play a role in motivation and aggression.
1. Pleasure and Reward: Dopamine is often referred to as the "feel-good" neurotransmitter because it is associated with feelings of pleasure and reward. When you engage in activities that are pleasurable or rewarding, such as eating a delicious meal or receiving positive feedback, dopamine is released in certain brain regions, such as the nucleus accumbens. This release of dopamine reinforces the behavior and encourages you to repeat it.
2. Motivation: Dopamine is closely linked to motivation. It can drive you to pursue goals and take actions to obtain rewards. When you anticipate a pleasurable outcome, such as achieving a personal goal or receiving a reward, dopamine levels in the brain increase, which can boost your motivation to work towards that outcome.
3. Mood Regulation: Dopamine also plays a role in regulating mood. Abnormal dopamine levels or signaling in the brain have been associated with mood disorders such as depression and bipolar disorder. An imbalance in dopamine activity can contribute to changes in mood and emotional states.
4. Attention and Focus: Dopamine is involved in attention and concentration. Optimal dopamine levels in the prefrontal cortex help maintain focus and attention on tasks. Conditions like attention deficit hyperactivity disorder (ADHD) are characterized, in part, by dysregulation of dopamine in these brain regions.
5. Learning and Memory: Dopamine is important for learning and memory processes. It helps strengthen the connections between neurons, allowing you to remember experiences and learn from them. This reinforcement of neural connections is essential for adapting behavior based on past experiences.
6. Addiction: Dopamine plays a central role in addiction. Substance abuse and addictive behaviors, such as gambling, can lead to excessive dopamine release in the reward pathways of the brain. Over time, this can lead to cravings and compulsive behaviors as individuals seek to experience the pleasurable effects associated with increased dopamine.
7. Risk-taking and Impulsivity: Dopamine has been linked to risk-taking behavior and impulsivity. Elevated dopamine levels can lead to a willingness to take risks and make impulsive decisions, sometimes with negative consequences.
It's important to note that dopamine is just one of many neurotransmitters and factors that influence human emotions and behavior. Its effects are part of a complex interplay of neurochemistry, genetics, environment, and individual differences. Moreover, excessive or dysregulated dopamine activity can contribute to various neuropsychiatric disorders.
While dopamine plays a significant role in emotions and behavior, it is just one piece of the puzzle, and understanding the broader context of brain function is essential for a comprehensive understanding of human behavior and emotional experiences.
Dopamine production in the brain is regulated through a complex process involving various enzymes, receptors, and feedback mechanisms.
Dopamine is a neurotransmitter that plays a crucial role in several physiological functions, including mood regulation, movement control, and the reward system. Here's an overview of how dopamine production is produced and regulated:
1. Precursor molecules: Dopamine is synthesized from precursor molecules, primarily the amino acid tyrosine. Tyrosine is obtained from dietary protein and is transported into brain cells, where it serves as the starting point for dopamine synthesis.
2. Tyrosine hydroxylase: The rate-limiting step in dopamine production is the conversion of tyrosine to L-dihydroxyphenylalanine (L-DOPA), which is catalyzed by the enzyme tyrosine hydroxylase. This enzyme requires oxygen, tetrahydrobiopterin (BH4), and iron as cofactors for its activity. Tyrosine hydroxylase is regulated through feedback mechanisms that control its activity based on the levels of dopamine and other neurotransmitters in the brain.
3. L-DOPA decarboxylase: L-DOPA is then converted into dopamine by another enzyme called L-DOPA decarboxylase (also known as aromatic L-amino acid decarboxylase). This enzyme removes a carboxyl group from L-DOPA to form dopamine.
4. Storage in vesicles: Dopamine is transported into synaptic vesicles in the presynaptic neuron by a protein called vesicular monoamine transporter 2 (VMAT2). Inside these vesicles, dopamine is stored until it is released into the synapse during neurotransmission.
5. Release and reuptake: When a nerve impulse reaches the presynaptic neuron, it triggers the release of dopamine into the synaptic cleft (the small gap between neurons). Dopamine molecules then bind to receptors on the postsynaptic neuron, transmitting the signal. Excess dopamine is removed from the synaptic cleft through reuptake by dopamine transporters (DAT) on the presynaptic neuron. Drugs like cocaine and amphetamines can interfere with dopamine reuptake and lead to increased dopamine levels in the synapse.
6. Regulation through feedback mechanisms: Dopamine production is tightly regulated by feedback mechanisms to maintain the appropriate levels in the brain. When dopamine levels are high, it can inhibit the activity of tyrosine hydroxylase, reducing the production of L-DOPA and dopamine. Conversely, when dopamine levels are low, this inhibition is reduced, allowing for increased dopamine synthesis.
7. Degradation: Dopamine can be broken down by enzymes such as monoamine oxidase (MAO) and catechol-O-methyltransferase (COMT) to produce metabolites that are eventually excreted from the body.
Various factors, including genetics, diet, and drugs, can influence dopamine production and regulation. Dysregulation of the dopamine system is associated with a range of neurological and psychiatric disorders, such as Parkinson's disease, schizophrenia, and addiction. Medications that affect dopamine levels are commonly used in the treatment of these conditions to help restore balance in the brain.
DOPAMINE
DOPAMINE REFERENCES
https://www.youtube.com/watch?v=1WZwzy9rCDk&t=483s
https://medicalxpress.com/news/2023-02-neural-substrate-modulate-sex-differences.html
https://www.youtube.com/watch?v=SoBuDctENTw
https://www.youtube.com/watch?v=QmOF0crdyRU&t=167s
https://www.sciencealert.com/key-hormone-dopamine-could-set-us-apart-from-apes
https://baos.pub/dopamine-nation-2090dfd7d74b
https://www.youtube.com/watch?v=UVPosGIUkUk
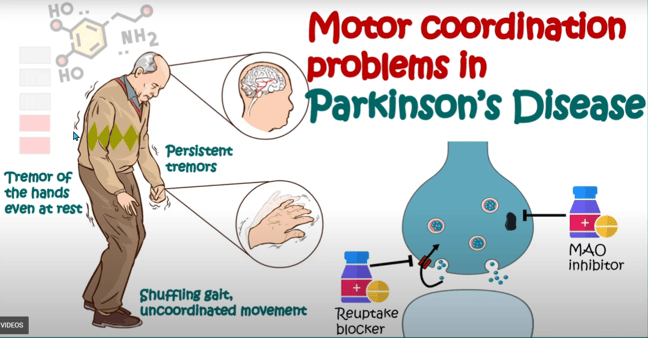
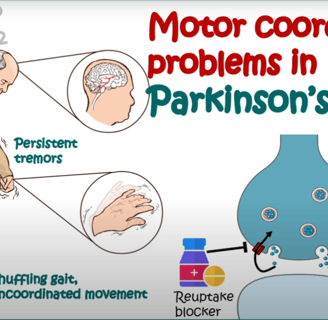
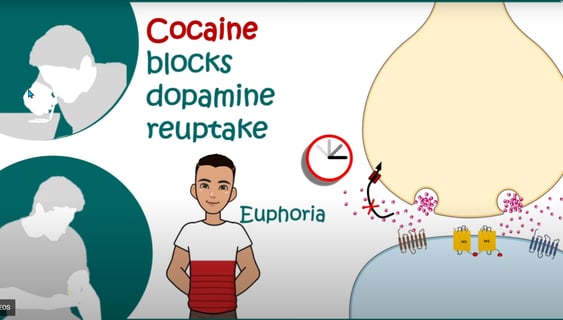
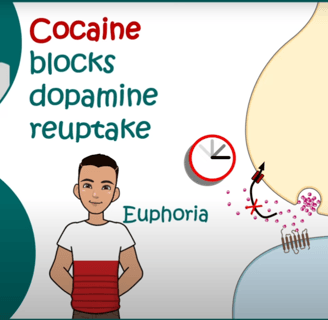
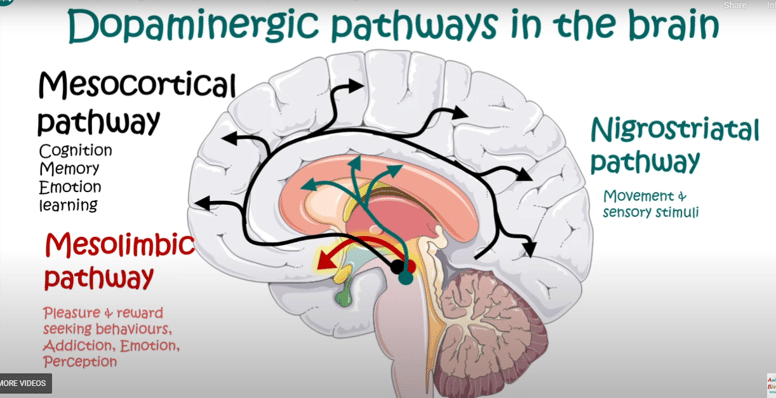
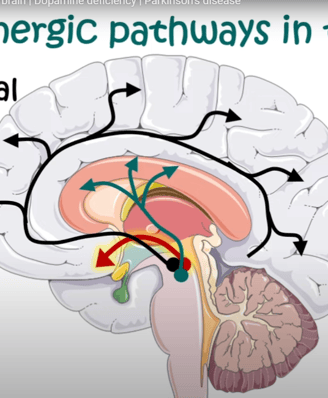
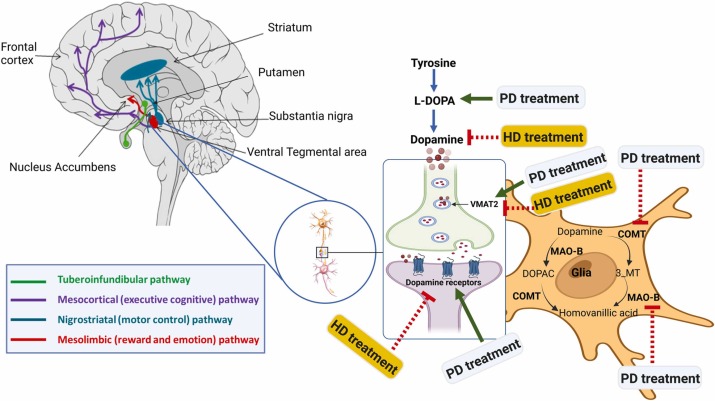
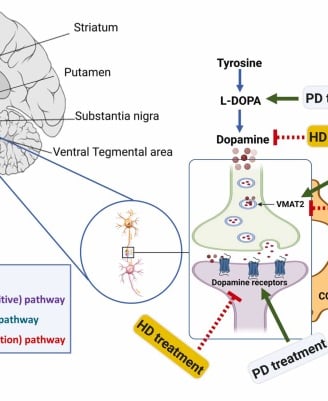
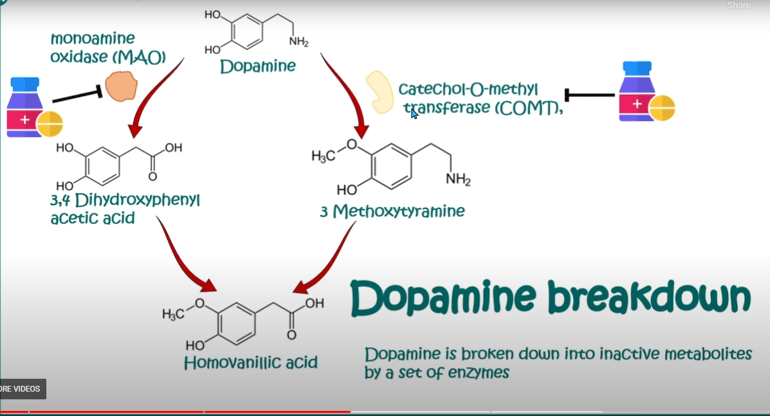
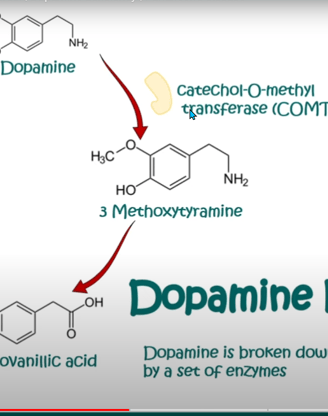
Brain-Derived Neurotrophic Factor (BDNF)
Brain-derived neurotrophic factor (BDNF) is a protein essential for the growth, maintenance, and survival of neurons in the brain and nervous system. It is a part of the neurotrophin family of proteins, which are responsible for promoting the survival, growth, and differentiation of neurons. Here’s a detailed look at the multifaceted role of BDNF:
BDNF as a Neurotrophic Factor
BDNF acts as a neurotrophic factor, meaning it supports the health and functioning of neurons. It promotes the survival of existing neurons and encourages the growth and differentiation of new neurons. This is particularly important for the plasticity and adaptability of the nervous system.
- Neurogenesis: BDNF is critical for neurogenesis, the process of forming new neurons. It supports the survival of new neurons in the hippocampus, a brain region essential for learning and memory.
- Maintenance: BDNF ensures the long-term health of neurons, protecting them from damage and degeneration.
Enhancing Synaptic Plasticity
Synaptic plasticity refers to the ability of synapses (the connections between neurons) to change and adapt in response to experience and learning. BDNF is crucial for this process:
- Long-Term Potentiation (LTP): BDNF strengthens synapses during LTP, which enhances the ability of neurons to communicate more effectively. This is a fundamental process for learning and memory consolidation.
- Long-Term Depression (LTD): BDNF also plays a role in LTD, where synaptic connections are weakened. This process is important for synaptic pruning and the removal of less active synaptic connections, which helps refine neural circuits.
Promoting Structural Plasticity
BDNF is involved in structural plasticity, which includes the physical remodeling of neurons and their connections:
- Dendritic Growth: BDNF promotes the growth and branching of dendrites, which are the parts of neurons that receive signals from other neurons. This dendritic growth is crucial for increasing the surface area available for synaptic connections.
- Synaptogenesis: BDNF stimulates the formation of new synapses, enhancing the brain’s ability to process and store information.
Influence of Lifestyle and Environmental Factors
BDNF levels can be significantly influenced by lifestyle choices and environmental factors:
- Physical Exercise: Regular physical activity has been shown to increase BDNF levels in various brain regions, including the hippocampus and cortex. Exercise promotes overall brain health and improves cognitive functions.
- Mental Stimulation: Engaging in mentally stimulating activities, such as learning new skills, reading, and solving puzzles, can boost BDNF production.
- Enriched Environments: Exposure to enriched environments, which provide a variety of sensory, cognitive, and social stimuli, can enhance BDNF levels and support neuroplasticity.
Neuroprotection and Health
BDNF not only supports neuron growth but also protects neurons from damage and degeneration:
- Neuroprotection: BDNF shields neurons from oxidative stress, excitotoxicity, and inflammation, which are common causes of neuronal damage. This protective role is crucial for maintaining a healthy neural network.
- Mental Health: Adequate levels of BDNF are associated with better mental health. Low levels of BDNF have been linked to various psychiatric disorders, including depression, anxiety, and schizophrenia.
BDNF in Brain Development
During early development, BDNF is essential for brain growth and the formation of neural circuits:
- Guidance of Neurons: BDNF guides the growth of neurons to their correct targets, ensuring proper neural circuit formation.
- Synaptic Refinement: BDNF helps refine synaptic connections, ensuring efficient and precise communication between neurons.
BDNF in Neurological and Psychiatric Disorders
Dysregulation of BDNF has been implicated in several neurological and psychiatric conditions:
- Alzheimer’s Disease: Reduced BDNF levels have been observed in Alzheimer’s patients. Enhancing BDNF signaling is being explored as a potential therapeutic approach to slow cognitive decline.
- Depression: Lower levels of BDNF are linked to depression. Antidepressant treatments often aim to increase BDNF levels to improve mood and cognitive function.
- Schizophrenia: BDNF dysregulation is associated with schizophrenia, affecting cognitive functions and neural connectivity.
Factors Influencing BDNF Production
- Electrical Activity: Increased neuronal activity, such as high-frequency stimulation, can trigger BDNF release.
- Neuropeptides: Signaling molecules like neurotrophins and calcitonin gene-related peptide (CGRP) influence BDNF release.
- Caffeine and Cinnamon: Studies suggest that caffeine and cinnamon (through its metabolite sodium benzoate) may boost BDNF levels.
Summary
BDNF is a critical protein that supports the growth, survival, and functioning of neurons in the brain and nervous system. Its roles in synaptic and structural plasticity make it vital for learning, memory, and adaptation to new experiences. Lifestyle choices, such as exercise and mental stimulation, can enhance BDNF production, promoting better brain health. Researchers continue to study BDNF to uncover its potential therapeutic applications for treating various neurological and psychiatric conditions.
Serotonin is often associated with mood regulation, and imbalances in serotonin levels can influence aggression. primarily found in the brain and gastrointestinal tract
-Low serotonin levels have been linked to increased impulsivity and aggressive behaviors in some studies.
Serotonin is often associated with mood regulation. Imbalances in serotonin levels have been linked to mood disorders like depression and anxiety. Medications like SSRIs (Selective Serotonin Reuptake Inhibitors) are commonly used to modulate serotonin levels.Serotonin, also known as 5-hydroxytryptamine (5-HT), is a neurotransmitter in the human body that plays a crucial role in regulating mood, emotion, sleep, and various physiological functions. The production and regulation of serotonin involve a complex process that occurs in several steps. Here's an overview of how serotonin is produced and regulated in humans:
1. Precursor Molecule: Serotonin is synthesized from a precursor molecule called tryptophan, which is an essential amino acid obtained from the diet. Tryptophan is transported into the brain, where it serves as the starting point for serotonin synthesis.
2. Tryptophan Hydroxylase: The rate-limiting step in serotonin production is the conversion of tryptophan into 5-hydroxytryptophan (5-HTP), which is catalyzed by the enzyme tryptophan hydroxylase. This enzyme requires oxygen and the cofactor tetrahydrobiopterin (BH4) for its activity. There are two isoforms of tryptophan hydroxylase, with the second one, known as TPH2, being primarily responsible for serotonin synthesis in the brain.
3. Aromatic L-amino Acid Decarboxylase (AADC): After the conversion of tryptophan into 5-HTP, AADC catalyzes the decarboxylation of 5-HTP, leading to the formation of serotonin.
4. Storage in Vesicles: Once synthesized, serotonin is transported into synaptic vesicles in neurons by the vesicular monoamine transporter (VMAT2). This storage helps maintain a reserve of serotonin for release during neurotransmission.
5. Release and Reuptake: When a nerve impulse reaches the presynaptic neuron, it triggers the release of serotonin into the synaptic cleft (the gap between neurons). Serotonin molecules then bind to receptors on the postsynaptic neuron, transmitting the signal. Excess serotonin is removed from the synaptic cleft through reuptake by serotonin transporters (SERT) on the presynaptic neuron, similar to how dopamine is reuptaken.
6. Regulation through Feedback Mechanisms: Serotonin production is tightly regulated by feedback mechanisms to maintain appropriate levels in the brain. Factors such as the availability of tryptophan and the activity of tryptophan hydroxylase can influence the rate of serotonin synthesis. Additionally, serotonin itself can inhibit its own synthesis by negatively regulating tryptophan hydroxylase.
7. Metabolism and Breakdown: Serotonin is eventually metabolized in the liver and other tissues by the enzyme monoamine oxidase (MAO), leading to the formation of 5-hydroxyindoleacetic acid (5-HIAA), which is then excreted in urine.
The regulation of serotonin levels in the brain is crucial for mood stability and overall emotional well-being. Imbalances in serotonin activity are associated with various psychiatric conditions, including depression, anxiety disorders, and some forms of schizophrenia.
Medications like selective serotonin reuptake inhibitors (SSRIs) are commonly used to increase serotonin levels in the brain and are often prescribed to treat mood disorders.
It's important to note that the serotonin system is highly complex, and its regulation involves multiple factors, including genetic predisposition, environmental influences, and interactions with other neurotransmitters and signaling pathways.
HOW DOES SERATONIN AFFECT HUMAN EMOTIONS AND BEHAVIOR
Key ways in which serotonin affects human emotions and behavior:
1. Mood Regulation: Serotonin is often referred to as the "feel-good" neurotransmitter because it is associated with a positive mood and emotional well-being. Adequate serotonin levels in the brain are thought to contribute to a stable and positive mood, while low levels have been linked to mood disorders such as depression and anxiety.
2. Depression and Anxiety: Serotonin deficiency is often associated with mood disorders, particularly depression and anxiety. Medications like selective serotonin reuptake inhibitors (SSRIs) are commonly used to increase serotonin levels in the brain and are effective in treating these conditions for many individuals. By increasing serotonin availability in the synaptic cleft, SSRIs can help improve mood and reduce symptoms of depression and anxiety.
3. Sleep Regulation: Serotonin is involved in regulating sleep patterns and the sleep-wake cycle. It helps promote wakefulness during the day and is converted into melatonin at night, which plays a role in initiating and maintaining sleep.
4. Aggression and Impulsivity: There is evidence to suggest that serotonin may modulate aggressive and impulsive behavior. Low serotonin levels have been associated with increased impulsivity and a greater likelihood of aggressive behavior. Some studies have shown that altering serotonin levels in the brain can influence these behaviors.
5. Appetite and Satiety: Serotonin also plays a role in regulating appetite and satiety. It is involved in signaling feelings of fullness and satisfaction after eating. Some medications that affect serotonin receptors are used to help with weight management by reducing food cravings.
6. Social Behavior: Serotonin has been implicated in social behavior and interactions. It may influence how individuals perceive and respond to social cues, impacting social bonding, cooperation, and empathy.
7. Learning and Memory: Serotonin is involved in learning and memory processes, although its role is complex and not fully understood. Some studies have suggested that serotonin can modulate memory formation and cognitive functions.
8. Pain Perception: Serotonin can influence pain perception and tolerance. It may play a role in pain modulation and contribute to the perception of pain intensity.
It's important to note that serotonin's effects on human emotions and behavior are highly context-dependent and can vary among individuals. Moreover, serotonin functions within a broader neurochemical system that includes other neurotransmitters and signaling pathways, making it just one component of the complex mechanisms that regulate emotions and behavior.
Individual differences in serotonin function, genetics, and environmental factors all contribute to how serotonin affects a person's emotional and behavioral responses.
Dysregulation of serotonin signaling is associated with various neuropsychiatric disorders, emphasizing the importance of maintaining balanced serotonin levels for emotional well-being and mental health.
Serotonin
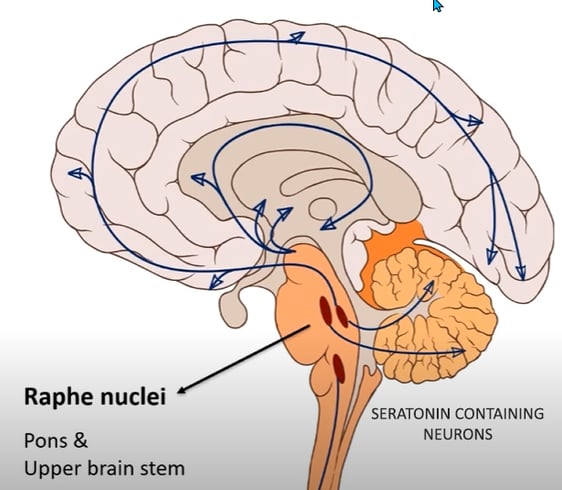
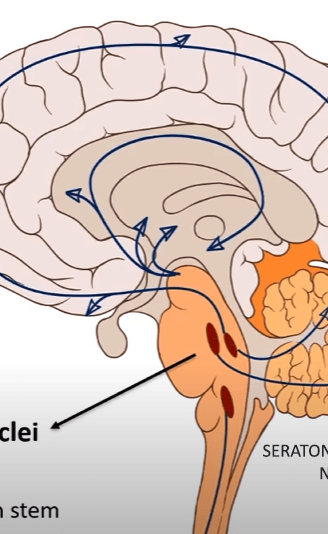
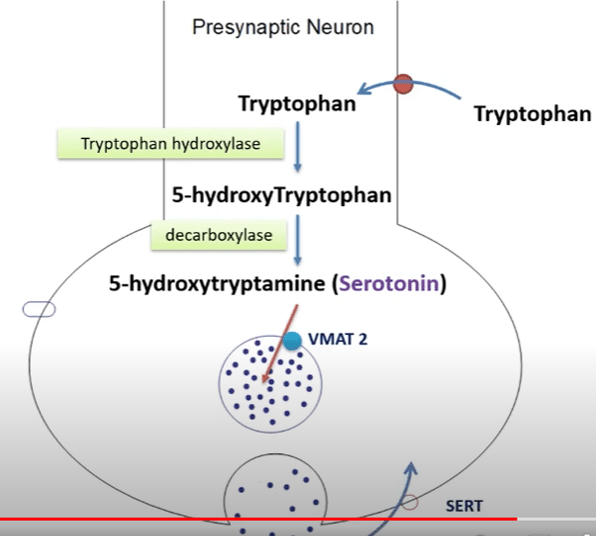
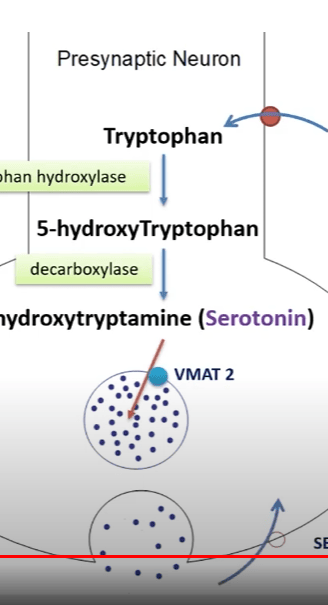
PRODUCTION
PATHWAYS
When many people think of someone with a high level of testosterone, they may picture a man loaded with strength, sexual prowess, and machismo. But while high-T has been correlated with all those things, it's also been correlated with status-seeking, dominance, aggression, sexual misconduct, and violence.
In some cases, high testosterone levels can cause mood changes, including increased irritability or aggression. Changes in sexual function and libido may occur. For people with a vulva, high testosterone levels can lead to an irregular menstrual cycle, increased muscle mass, or an enlarged clitoris.Testosterone is only able to enhance the mood of people with low testosterone, and cannot alleviate depressive symptoms in non-hypogonadal populations. However, men with testosterone deficiency may feel less depressed and have more zest for life after TRT.Testosterone increases the release of dopamine, which is a neurotransmitter in your brain responsible for your feelings of pleasure. There may also be a connection between testosterone levels and the function of serotonin. A decrease in serotonin activity in the brain is linked to feelings of depression. Do endorphins increase testosterone?
Furthermore, we have demonstrated that acute or chronic beta-endorphin treatment does not affect testosterone production by Leydig cells in vitro, consistent with the absence of receptors on these cells.Yes, testosterone can affect endorphins. In one study, castrating male rats for one week significantly decreased the release of beta-endorphin and dynorphin from hypothalamic slices. However, when testosterone was administered to the rats in increasing doses, the release rates increased in a dose-dependent manner. After four weeks of castration, the release rates of the rats were similar to those of intact rats, suggesting that castration initially affects both the release and synthesis of opioid peptides, but that release eventually normalizes while synthesis remains elevated.
Significance of testosterone in regulating hypothalamic ...Basal in vitro release rates of beta-endorphin and dynorphin were significantly lower from hypothalamic slices derived from 1-week castrated animals than from intact males, and when testosterone was administered in various doses in vivo, basal release rates in vitro increased in a dose-related manner. Hypothalami from rats that had been castrated for 4 weeks, however, showed basal release rates similar to those in tissues from intact controls, a finding indicating that castration initially alters both opioid peptide synthesis and release; later, release is normalized, whereas synthesis remains elevated.Testosterone levels in healthy men correlate negatively with serotonin 4 receptor binding
The serotonergic system integrates sex steroid information and plays a central role in mood and stress regulation, cognition, appetite and sleep. This interplay may be critical for likelihood of developing depressive episodes, at least in a subgroup of sensitive individuals.
The serotonin 4 receptor (5-HT4R) indexes central serotonergic tonus, which may be related to endogenous sex-steroid levels in the mentally healthy state even though this remains elusive. Here we evaluate if peripheral levels of estradiol and testosterone are associated with 5-HT4R binding as imaged by [11C]SB207145 positron emission tomography in a group of 41 healthy men. We estimated global 5-HT4R binding using a latent variable model framework, which models shared correlation between 5-HT4R across multiple brain regions (hippocampus, amygdala, posterior and anterior cingulate, thalamus, pallidostriatum and neocortex). We tested whether testosterone and estradiol predict global 5-HT4R, adjusting for age.
We found that testosterone, but not estradiol, correlated negatively with global 5-HT4R levels (p = 0.02) suggesting that men with high levels of testosterone have higher cerebral serotonergic tonus. Our findings corroborate the link between sex hormone levels and serotonin signalling. Future longitudinal studies in clinical relevant populations are needed to elucidate the potential importance of testosterone in the pathophysiology of e.g. major depression and its treatment Testosterone is a hormone that can influence aggression, particularly in males. Higher levels of testosterone have been linked to increased aggressive behaviors in some studies.Testosterone is the primary male hormone responsible for regulating sex differentiation, producing male sex characteristics, spermatogenesis, and fertility.
Testosterone’s effects are first seen in the fetus. During the first 6 weeks of development, the reproductive tissues of males and females are identical. At around week 7 in utero, the SRY (sex-related gene on the Y chromosome) initiates the development of the testicles. Sertoli cells from the testis cords (fetal testicles) eventually develop into seminiferous tubules. Sertoli cells produce a Mullerian-inhibiting substance (MIS), which leads to the regression of the Fallopian tubes, uterus, and upper segment of the vagina (Mullerian structures normally present in females). Fetal Leydig cells and endothelial cells migrate into the gonad and produce testosterone, which supports the differentiation of the Wolffian duct (mesonephric duct) structures that go on to become the male urogenital tract. Testosterone also gets converted to dihydrotestosterone (DHT) in the periphery (discussed below) and induces the formation of the prostate and male external genitalia. Testosterone is also responsible for testicular descent through the inguinal canal, which occurs in the last 2 months of fetal development. When an embryo lacks a Y chromosome and thus the SRY gene, ovaries develop. Fetal ovaries do not produce adequate amounts of testosterone, thus the Wolffian ducts do not develop. There is also an absence of MIS in these individuals, leading to the development of the Mullerian ducts and female reproductive structures. [1]
Function
Testosterone is responsible for the development of primary sexual development, which includes testicular descent, spermatogenesis, enlargement of the penis and testes, and increasing libido. The testes usually begin the descent into the scrotum around 7 months of gestation, when the testes begin secreting reasonable quantities of testosterone. If a male child is born with undescended but normal testes that do not descend by 4 to 6 months of age, administration of testosterone can help the testes descend through the inguinal canals. [2]
Testosterone is also involved in regulating secondary male characteristics, which are those responsible for masculinity. These secondary sex characteristics include male hair patterns, vocal changes, and voice deepening, anabolic effects, which include growth spurts in puberty (testosterone increases tissue growth at the epiphyseal plate early on and eventual closure of plate later in puberty) and skeletal muscle growth (testosterone stimulates protein synthesis). Testosterone also stimulates erythropoiesis, which results in a higher hematocrit in males versus females. Testosterone levels tend to drop with increasing age; because of this, men tend to experience a decrease in testicular size, a drop in libido, lower bone density, muscle mass decline, increased fat production, and decreased erythropoiesis, which leads to possible anemia.
Mechanism
In puberty, the hypothalamic-pituitary-gonadal axis plays a major role in regulating testosterone levels and gonadal function. The hypothalamus secretes GnRH, which travels down the hypothalamohypophyseal portal system to the anterior pituitary, which secretes luteinizing hormone (LH) and follicle-stimulating hormone (FSH). LH and FSH are two gonadotropic hormones that travel through the blood and act on receptors in the gonads. LH, in particular, acts on the Leydig cells to increase testosterone production. Testosterone limits its own secretion via negative feedback. High levels of testosterone in the blood feedback to the hypothalamus to suppress the secretion of GnRH and also feedback to the anterior pituitary, making it less responsive to GnRH stimuli. [3]
Throughout the reproductive life of males, the hypothalamus releases GnRH in pulses every 1 to 3 hours. Despite this pulsatile release, however, average plasma levels of FSH and LH remain fairly constant from the start of puberty, where levels spike, to the third decade of life, where levels peak and slowly begin to decline. Prior to puberty, testosterone levels are low, reflecting the low secretion of GnRH and gonadotropins. Changes in neuronal input to the hypothalamus and brain activity during puberty cause a dramatic rise in GnRH secretion.
Leydig cells in the testes function to turn cholesterol into testosterone. LH regulates the initial step in this process. Two important intermediates in this process are dehydroepiandrosterone (DHEA) and androstenedione. Androstenedione is converted to testosterone by the enzyme 17-beta-hydroxysteroid dehydrogenase. The majority of testosterone is bound to plasma proteins such as sex-hormone-binding-globulin and albumin. This majority supply of protein-bound testosterone acts as a surplus of testosterone hormone for the body. The small amounts of free testosterone in the blood act at the level of the tissues, primarily the seminal vesicles, bone, muscle, and prostate gland. At the cellular level, testosterone gets converted to dihydrotestosterone by the enzyme 5-alpha-reductase. Testosterone and dihydrotestosterone can bind to cell receptors and regulate protein expression. Both men and women also produce weak acting androgens in the zona reticularis of the adrenal glands. These weak-acting androgens are known as dehydroepiandrosterone and androstenedione. They bind to testosterone receptors with weaker affinity but can also be converted to testosterone in the peripheral tissues if produced at high amounts. [4]
Related Testing
Features of testosterone deficiency can be very apparent, which is why the first steps in diagnosing male hypogonadism involve adequate history taking and physical exam. The features indicative of male hypogonadism can be divided into pre and post-pubertal. Pre-pubertal features include small testes (less than 20 mL in volume), small phallus, decreased secondary sex characteristics (e.g., facial or axillary hair), gynecomastia, difficulty gaining muscle mass, eunuchoid proportions, low sperm count, and low energy/libido. Post-pubertal features include those previously mentioned (except phallus size and eunuchoid proportions) as well as osteoporosis and hot flashes with severe hypogonadism.
If a clinician expects hypogonadism based on history and physical, a total serum testosterone between 8 AM and 10 AM should be drawn. Normal levels may indicate eugonadal low testosterone. If levels are low, a repeat level should be obtained along with FSH and LH levels. Low testosterone in the setting of normal FSH/LH indicates secondary hypogonadism. The next steps would be to get prolactin, T4, 8 AM cortisol, iron, and ferritin levels as well as brain MRI. Low testosterone in the setting of elevated FSH/LH indicated primary hypogonadism. In the case of primary hypogonadism, a karyotype should be established.
Hyperandrogenism also has various clinical presentations, depending on puberty status and gender. Prepubertal boys with hyperandrogenism may present with virilization. Virilization includes penile enlargement, excess hair growth in androgen-dependent areas, and voice deepening. In prepubertal girls, hyperandrogenism may lead to clitoromegaly, acne, and hirsutism. In adult males, the effects of excess testosterone depend on whether the source is from the adrenals or exogenous. Adrenal androgen elevations have few observable effects in males and do not cause an increase in muscle mass or hair growth. In adult females, increased adrenal androgens can lead to acne, hirsutism, menstrual irregularities, infertility, male-pattern baldness, or virilization.
Testosterone can be used to treat and manage various medical conditions. Medical conditions in which testosterone can be used include metastatic breast cancer, delayed puberty, hypogonadotropic hypogonadism (congenital or acquired), and primary hypogonadism. Toxic effects of testosterone and synthetic androgens include over-masculinization, hirsutism, decreased menses, acne, and clitoral enlargement. Rarely, synthetic androgens can cause hepatic adenoma, cholestatic jaundice, and prostatic hypertrophy. Synthetic androgens and testosterone are contraindicated in pregnancy.
Androgen antagonists come in different types. GnRH analogs, if given continuously, can act as medical castration drugs and are used in treating prostate cancer. Androgen receptor inhibitors, like flutamide and spironolactone, can be used for patients with hirsutism. Steroid synthesis inhibitors, like ketoconazole, can be used in Cushing disease. 5-alpha reductase inhibitors, like finasteride, can be used to treat benign prostatic hyperplasia. [5]
Clinical Significance
Pathology related to testosterone involves either over-production, under-production, receptor insensitivity, or impaired metabolism of testosterone. The following are a few of the more common and highly tested testosterone pathologies.
Over-production of androgens can occur in the following conditions: polycystic ovarian syndrome (PCOS), adrenal virilization/adrenal tumors, ovarian or testicular tumors, Cushing syndrome, and as a result of exogenous steroid use. To better understand some of these pathologies it is important to note the differences between testosterone and dehydroepiandrosterone (DHEA). DHEA is a relatively weak androgen produced by the adrenals and ovaries/testes. DHEA serves as a precursor for other hormones including testosterone and estrogen. The sulfated form of DHEA, DHEAS, is specific for the adrenal glands. In polycystic ovary syndrome (PCOS), abnormal gonadotropin-releasing hormone (GnRH) secretion leads to an increase in LH secretion. LH stimulates androgen production by ovarian theca cells which leads to hirsutism, male escutcheon, acne and androgenic alopecia in women affected with PCOS.[6] In adrenal and ovarian tumors, there is usually rapidly progressing androgenic symptoms (hirsutism, virilization). If testosterone is elevated and DHEAS is normal, this is most likely from an ovarian tumor. If DHEAS is elevated and testosterone is relatively normal, this is most likely an adrenal tumor.
Decreased production of testosterone can occur with aging, certain medications, chemotherapy, hypothalamus-pituitary axis disorders, primary hypogonadism, cryptorchidism and orchitis, and with genetic disorders such as Klinefelter and Kallmann syndrome. Klinefelter syndrome is the most common congenital abnormality that results in primary hypogonadism. In Klinefelter, there is dysgenesis of seminiferous tubules and loss of Sertoli cells which leads to a decrease in inhibin levels and a resultant increase in FSH. FSH upregulates aromatase leading to increased conversion of androgens to estrogens. In Klinefelter, there is also Leydig cell dysfunction which leads to decreased testosterone levels and an increase in LH due to loss of negative feedback. In Kallmann syndrome, failed migration of GnRH-producing neurons leads to lack of GnRH. No GnRH results in a decrease in LH, FSH, testosterone, and sperm count. Specific to Kallmann syndrome, in comparison to other causes of hypogonadotropic hypogonadism, is defects in the sensation of smell (hyposmia or anosmia).
5-alpha reductase is an enzyme that converts testosterone to dihydrotestosterone. Male patients with 5-alpha reductase deficiency present with normal female or male genitalia or ambiguous genitalia at birth due to lack of dihydrotestosterone. These patients have a male internal urogenital tract (anti-Mullerian hormone is still present). At puberty, adolescents with this enzyme deficiency, who may have been raised as girls due to lack of secondary male characteristics, begin to develop male secondary sex characteristics and have primary amenorrhea. These patients will have normal testosterone and LH, low DHT, and an increased testosterone-to-DHT ratio. In contrast to 5-alpha reductase deficiency, androgen insensitivity is a condition in which patients lack functional androgen receptors resulting in under-virilization. These patients, like those with 5-alpha reductase deficiency, have a 46 XY karyotype. In contrast, however, these patients have normal female external genitalia and usually undescended testes. In adolescence, they experience primary amenorrhea and breast development but have no pubic or axillary hair and lack the deepening voice changes that occur with puberty. They will have a blind vaginal pouch and abnormal internal reproductive organs (fallopian tubes, uterus, and the upper portion of the vagina) due to the production of the Mullerian inhibiting factor. These patients will have high levels of testosterone and LH.
Impaired testosterone metabolism can occur in certain cases of congenital adrenal hyperplasia (CAH). In classic CAH (95% of cases), due to 21 hydroxylase deficiency, newborns usually present with ambiguous genitalia and later develop salt wasting, vomiting, hypotension, and acidosis. A marked increase in 17-hydroxyprogesterone is diverted towards adrenal androgen synthesis and leads to hyperandrogenism. Hyperandrogenism impairs hypothalamic sensitivity to progesterone leading to a rapid rise in GnRH synthesis and thus increased LH and FSH. Elevations in LH and FSH lead to increased gonadal steroid production (17-hydroxyprogesterone, DHEA, testosterone, LH, and FSH). Diagnosis is with adrenocorticotropic hormone stimulation test showing exaggerated 17 hydroxyprogesterone response. [11]
TESTOSTERONE..
TESTOSTERONE REFERERENCES
GABA (Gamma-Aminobutyric Acid)
Glutamate
GABA is an inhibitory neurotransmitter that helps regulate anxiety and stress by reducing neuronal excitability. Drugs that enhance GABA's effects are sometimes used to treat anxiety disorders.
Glutamate is the primary excitatory neurotransmitter in the brain and is involved in many aspects of brain function, including learning and memory. Dysregulation of glutamate can be associated with conditions like epilepsy and certain mood disorders.
EXCERPTS FROM A PHYCHIATRIST TALKS ABOUT NEUROTRANSMITTER GABA-GLUTAMATE BALANCE
GLUTAMATE IS YOUR EXCITORY NEUROTRANSMITTER - you feel up it makes you feel awake and ready and epinephrine of course plays a role , day-to-day glutamate is more important
Gaba is a neurotransmitter and most people have heard of the Superstar neurotransmitters I but 80% of your neurotransmission has nothing to do with those neurotransmitters at all
it's a balance between two
it's a balance between glutamate and Gaba -
glutamate actually turns into Gaba in the brain
Gaba is not made really anywhere else except for a very small amount in the pancreas - Gaba is made in the brain and Gaba is responsible for 20% of your brain's neurotransmission, 60% is from the gut so that's a total of 80%
GABA IS YOUR NEUROTRANSMITTER THAT'S RESPONSIBLE FOR RELAXATION FOR MAKING THE BRAIN RELAX so you need to have the balance between these two neurotransmitters to be able to be get excited and also to relax and to recover
if you don't have enough Gaba around you're going to feel irritable you're going to feel potentially a lot of different symptoms might call it it's called Gaba deficiency syndrome and s it could be things like depression ,anxiety ,stress ,poor sleep - could even be severe things like Tremors and difficulty urinating and difficulty with your emotions - irritability with your mood going up and down this can happen from a lot of different things - but people forget that Gaba could be one of those neurotransmitters that is depleted because all of us are so stressed all the time.depleting our stressed neurotransmitters depleting glutamate and not recovering enough to be able to get enough Gaba in the system so okay with that how does one deplete glutamate
if we have infection we deplete Gaba -if we have stress on the system we deplete it as well
glutamate is made from glutamine so glutamine is an amino acid d -well known to most people in the the bodybuilding performance - glutamine is also the fuel for the colon cells itself but glutamine gets converted in the body, to glutamate in the brain but- if you're deficient in glutamine mean you're not going to make enough glutamate for your brain - if you can't make enough glutamate then you can't make enough Gaba - if you can't make enough Gaba you can't relax the firing of your brain
with deficiency in glutamine - not getting enough protein in your diet - meat is going to be your biggest source here -y people that are vegan or vegetarian are going to have a much harder time getting enough glutamine in their diet
if you are a elite athlete and always training you're also probably going to have a leaky gut and your gut is going to be sucking up all your glutamine in your gut itself to try to help fuel it not a lot's going to get into your brain and make glutamate and then very little is going to get over to Gaba so you're going to be Gaba deficient you're not going to sleep well you're going to feel irritable all the time
to convert from glutamate to Gaba you need magnesium you need Vitamin B6 so if you're deficient in magnesium or deficient in vitamin B6 you're not going to convert glutamate to Gaba well .
Go to a Chinese restaurant and you immediately get a headache that's because you have suddenly a huge preponderance of glutamate compared to Gaba and as a result of that you have this headache that typically will happen as a result of that excitability that happens because of too much glutamate - really upsets people because I think that it's like their MSG
it doesn't contribute to muscle protein synthesis hardly at all so is there actually a practical app for taking a glutamine
Gaba-Cava root Cava plant from South the South Pacific has been around for thousands of years for stress for relaxation it's enhancing the Gaba system we also have something called agarin agarin is a it's a Gaba Agonist which means that it actually binds to the Gaba site itself and this is really cool -agarin from the amonita musara mushroom so it's a mushroom that's gotten very famous from Allison Wonderland and from mythology about Santa Claus and causing psychedelic experiences but it has two molecules in there in the mushroom it has something called agarin and has something called ibotenic acid ibotenic acid is neurotoxic that's what causes the Psychedelic experiences but this other one called agarin is a fantastic compound for sleep because- it's a long acting Gaba Agonist it stays on the Gaba receptor for a while it increases Gaba firing so you have this 8 to 10 hour hours of a halflife so you have a whole night where you can actually get good sleep
Pharmaceuticals that are well known to be have a very high affinity for the gab receptor that you need to avoid things like benzodiazapines and alcohol and actually bind to the Gaba receptor as well but the difference with those is that they're such a high affinity for the binding and their pH pharmacologic that you know there's a high risk for tolerance for withdrawal you're taking something that to enhance Gaba system you may want to take it at night because it may make you feel more relaxed it may make you feel more tired it may decrease stress -
Gaba supplements don't work if you take a Gaba supplement over the counter --Gaba itself is too big of a molecule to get across the blood brain barrier on its own so Gaba supplements don't typically work unless you have a leaky gut and that means you have a leaky brain people get scared when I say a leaky brain but all I mean there is that you have a leaky gut and so if people take Gaba supplements and they're feeling much more calm and relaxed on them it could mean that their gut is leaky and that their brain their blood brain barrier is not working as well.
Giving yourself the building blocks that are going to make Gaba right are you getting good sources of vitamin B6 in your diet are you getting good sources of magnesium in your diet I think like you know I was we were talking about before with you know being people being on this freight train uh and just having so much stress and not being able to calm down and like they're trying so hard with their nutrients and their diet but like it's really hard to find uh most people are magnesium deficient most people I think 80% of the US population I might be slightly wrong there but it's it's high it has some sort of element of magnesium deficiency and your vitamins and minerals like the foods that we're eating now about 20 or 30% of the amount of nutrients that they used to have 50 years ago
Taxing on our our dopamine compared to Gaba like do they do they seem to operate in tandem or things
Gaba is everywhere in the brain okay it's widely distributed in all areas of the brain and but specifically it has this one particular function in most locations it's called an inter neuron i n t r neuron which means it's between neurons
So it's actually between a lot of these serotonergic and dopamine dopaminergic neurons and regulating the flow of information so if you are massively taxing your dopamine system you're also taxing your Gaba system because your gab is trying to prevent the firing of all this stuff that you're looking at
Because dopamine and serotonin are being modulated by the Gaba system so gab is so important for learning for memory for skill acquisition um and of course what really important for skill acquisition but dopamine as well
But what Gaba does it actually tries to stop all the firing like and trying to regulate the information that's coming in so you actually can learn and so it's if you're depleting dopamine you're depleting Gaba
I work with the Special Forces operate - they teach them - it's not about amping up you don't see a lot of marksmen going forward and pounding caffeine right that's not how they want their brain to operate
Think if we could all kind of take a lesson from that - when you look at the things that make you feel good aren't always the things that are truly good for you
- Calms me down it's relaxing - there's a difference between something that is pleasure seeking behavior and what is relaxing - that's problem that they're going for that dopamine hit = my in my practice is relax control right the idea is that you're in control of the situation but you're relaxed into it right you're part of the situation but you're not because you're overstressed you're not going to be operating at the highest level that you want to be.
Oxytocin and Vasopressin are neuropeptides that have been implicated in social behaviors, including aggression. Oxytocin is often associated with prosocial behaviors, while vasopressin may play a role in aggressive and territorial behaviors.
Oxytocin is often referred to as the "love hormone" or "bonding hormone" because it plays a role in social bonding, attachment, and trust. It can influence emotional responses in social contexts.Oxytocin is a hormone and neuropeptide that plays a crucial role in various physiological processes and has a significant impact on the human body, particularly in the context of social and reproductive behaviors. Here is an overview of its production, functions, and effects on the human body:
Production
Oxytocin is primarily produced and released by the hypothalamus, a region of the brain. It is then transported and stored in the posterior pituitary gland, which releases oxytocin into the bloodstream when stimulated.
Several factors can trigger the release of oxytocin, including:
1. Childbirth: During labor and childbirth, oxytocin is released in large quantities. This hormone helps stimulate uterine contractions, facilitating the expulsion of the baby from the womb.
2. Breastfeeding: Oxytocin also plays a crucial role in lactation. It helps with the ejection of milk from the mammary glands, allowing infants to feed.
3. Social Bonding: Oxytocin is often referred to as the "love hormone" or "cuddle hormone" because it is released during intimate and social interactions, such as hugging, kissing, or cuddling.
4. Orgasm: Oxytocin levels in both men and women can increase during sexual arousal and peak at the time of orgasm. This hormone contributes to feelings of pleasure and emotional connection during sexual activity.
Effects on the Human Body
Oxytocin has a wide range of effects on the human body, both physiological and psychological:
1. Uterine Contractions: During labor, oxytocin stimulates the muscles of the uterus, leading to contractions. This is essential for the progression of labor and the eventual delivery of the baby.
2. Milk Ejection: In lactating mothers, oxytocin is responsible for the "let-down reflex," which allows milk to be released from the mammary glands, making it available for breastfeeding.
3. Social Bonding: Oxytocin plays a critical role in forming and strengthening social bonds. It enhances trust, empathy, and emotional connection between individuals, which is important for maintaining healthy relationships.
4. Stress Reduction: Oxytocin has been shown to reduce the body's stress response. It can help lower blood pressure and decrease levels of the stress hormone cortisol, promoting feelings of relaxation and well-being.
5. Sexual Function: Oxytocin is involved in sexual arousal and orgasm, contributing to feelings of pleasure and intimacy during sexual activity.
. Parental Behavior: In both mothers and fathers, oxytocin is associated with parental bonding and caregiving behaviors towards their children.
7. Social Recognition: Oxytocin may facilitate the recognition of familiar faces and the ability to perceive social cues and emotions in others.
It's important to note that oxytocin's effects can vary among individuals and may depend on various factors, including genetics, context, and individual sensitivity to the hormone. While oxytocin is often associated with positive social and emotional effects, its administration in high doses has also been studied in research contexts and is associated with potential side effects and complications, including social effects like increased trust in others and physiological effects like uterine contractions.
In summary, oxytocin is a multifaceted hormone with a range of effects on the human body, influencing social bonding, reproductive processes, and emotional well-being. Its role in promoting positive social interactions and emotional connections has garnered significant interest in various fields of research, including psychology, neuroscience, and endocrinology.
Oxytocin can interact with other neuromodulatory agents and neurotransmitters in the human body, influencing various physiological and behavioral processes. Here are some interactions and relationships between oxytocin and other neurotropic agents:
1. Dopamine: Oxytocin and dopamine often work together in the brain's reward and pleasure circuitry. The release of oxytocin during social interactions and bonding can lead to the release of dopamine, contributing to feelings of pleasure and reinforcement of social behaviors. This interaction plays a role in the positive emotions associated with social bonding, including love and attachment.
2. Serotonin: Oxytocin has been linked to serotonin, another neurotransmitter that regulates mood and social behavior. Some studies suggest that oxytocin can modulate serotonin levels in certain brain regions, potentially influencing mood and emotional regulation.
3. Endorphins: Oxytocin can also interact with endorphins, which are natural opioids produced by the body. The release of oxytocin during activities such as hugging, cuddling, and bonding can lead to the release of endorphins, contributing to feelings of pleasure and pain relief.
4. Vasopressin: Vasopressin is a related hormone to oxytocin and is involved in social behaviors, including pair bonding and aggression. Oxytocin and vasopressin often work together to regulate various aspects of social and reproductive behaviors, and their interactions can influence mating, territoriality, and social hierarchy.
5. Cortisol: Oxytocin has been shown to reduce the levels of cortisol, a stress hormone, in the body. This interaction suggests that oxytocin may have a role in reducing the physiological response to stress and promoting relaxation.
6. GABA (Gamma-Aminobutyric Acid): Some research suggests that oxytocin may enhance the effects of GABA, an inhibitory neurotransmitter in the brain. This interaction could contribute to oxytocin's calming and anxiolytic (anxiety-reducing) effects.
It's important to note that the interactions between oxytocin and these neurotropic agents are complex and context-dependent. They can vary based on individual differences, the specific brain regions involved, and the timing of hormone release. Research in this area is ongoing, and scientists are continually uncovering more about how oxytocin interacts with other neuromodulators to influence human behavior and physiology.
the use of exogenous oxytocin, such as in the form of medication or supplements, can have different effects and interactions compared to the natural release of oxytocin in the body, and the administration of oxytocin in research or clinical settings is subject to specific protocols and considerations.
Oxytocin
Vasopressin
Norepinephrine can be involved in the "fight or flight" response and may influence aggressive behaviors in certain situations. Norepinephrine is primarily involved in the "fight or flight" response and plays a role in arousal, attention, and alertness. It can influence mood and emotional responses.How does testosterone affect personality?
Norepinephrine (NE), also called noradrenaline (NA) or noradrenalin, is an organic chemical in the catecholamine family that functions in the brain and body as a hormone, neurotransmitter and neuromodulator. The name "noradrenaline" (from Latin ad, "near", and ren, "kidney") is more commonly used in the United Kingdom, whereas "norepinephrine" (from Ancient Greek ἐπῐ́ (epí), "upon", and νεφρός (nephrós), "kidney") is usually preferred in the United States.[2] "Norepinephrine" is also the international nonproprietary name given to the drug.[3] Regardless of which name is used for the substance itself, parts of the body that produce or are affected by it are referred to as noradrenergic.
The general function of norepinephrine is to mobilize the brain and body for action. Norepinephrine release is lowest during sleep, rises during wakefulness, and reaches much higher levels during situations of stress or danger, in the so-called fight-or-flight response. In the brain, norepinephrine increases arousal and alertness, promotes vigilance, enhances formation and retrieval of memory, and focuses attention; it also increases restlessness and anxiety. In the rest of the body, norepinephrine increases heart rate and blood pressure, triggers the release of glucose from energy stores, increases blood flow to skeletal muscle, reduces blood flow to the gastrointestinal system, and inhibits voiding of the bladder and gastrointestinal motility.
In the brain, noradrenaline is produced in nuclei that are small yet exert powerful effects on other brain areas. The most important of these nuclei is the locus coeruleus, located in the pons. Outside the brain, norepinephrine is used as a neurotransmitter by sympathetic ganglia located near the spinal cord or in the abdomen, as well as Merkel cells located in the skin. It is also released directly into the bloodstream by the adrenal glands. Regardless of how and where it is released, norepinephrine acts on target cells by binding to and activating adrenergic receptors located on the cell surface.
A variety of medically important drugs work by altering the actions of noradrenaline systems. Noradrenaline itself is widely used as an injectable drug for the treatment of critically low blood pressure. Stimulants often increase, enhance, or otherwise act as agonists of norepinephrine. Drugs such as cocaine and methylphenidate act as reuptake inhibitors of norepinephrine, as do some antidepressants, such as those in the SNRI class. One of the more notable drugs in the stimulant class is amphetamine, which acts as a dopamine and norepinephrine analog, reuptake inhibitor, as well as an agent that increases the amount of global catecholamine signaling throughout the nervous system by reversing transporters in the synapses.
Norepinephrine (noradrenaline)
Norepinephrine REFERENCES
https://pubmed.ncbi.nlm.nih.gov/2886553/
https://www.healthline.com/health/signs-of-high-testosterone
What is the function of the natural cortisol?
Cortisol is a stress hormone that can affect various aspects of behavior and physiology. Elevated cortisol levels in response to stress may influence survival instincts and decision-making.Endogenous cortisol levels may modulate amygdala functional connectivity with specific regions in the mPFC, even under relatively stress-free circumstances. Our results corroborate previous findings from both animal and human studies, suggesting cortisol-mediated regulation of the amygdala by the mPFC.Also known as the stress hormone, cortisol is produced by the adrenal glands and helps the body deal with stress by:
Mobilizing energy
Regulating your body's stress response: During times of stress, your body can release cortisol after releasing its “fight or flight” hormones, such as adrenaline, so you continue to stay on high alert. In addition, cortisol triggers the release of glucose (sugar) from your liver for fast energy during times of stress.
Maintaining fluid balance and blood pressureCortisol, often called the "stress hormone", can increase serotonin uptake in both humans and rabbits. Cortisol promotes the synthesis of the serotonin transporter, which can lead to a 37% increase in serotonin uptake. This increase in serotonin uptake can occur during rest and nerve stimulation, and may be a factor in depression associated with stress. However, some studies have found that people with chronic stress or depression may have reached the limit of their ability to increase serotonin transporters due to chronically elevated cortisol levels.
Enhancement of serotonin uptake by cortisol:
We report here that cortisol at the nM-microM concentration range induces a substantial increase in serotonin uptake both in vitro, by human peripheral blood lymphocytes (PBLs) and cortical neuronal cells, and in vivo, by rabbit PBLs, owing to promotion of synthesis of the serotonin transporter. These findings offer a novel molecular mechanism for depression associated with stress. Accordingly, the elevated cortisol induced by stress increases serotonin uptake, under both rest and nerve stimulation, which is overtly expressed in symptoms of depression.
Correlation between cortisol level and serotonin uptake
A significant increase in serotonin uptake (+37% + 14, M + SD) was observed in the control group, whereas neither the generalized anxiety disorder nor the major depression group exhibited changes in serotonin uptake upon incubation with cortisol. It is likely that under chronic stress or depression, the capacity for increase in serotonin transporter has reached its limit due to the chronically elevated blood cortisol level. The physiological and diagnostic implications of this observation are discussed.
CORTISOL
Endorphins
Endorphins are endogenous opioids that are released during exercise, excitement, and stress. They play a role in pain modulation and can induce feelings of euphoria.
Endorphins are naturally occurring chemicals in the human body that act as neurotransmitters and function as natural painkillers and mood elevators. They are produced in response to various stimuli and activities, primarily through the central nervous system. Here's a simplified explanation of how endorphins are produced and the chemical process involved:
1. Stimulus or Trigger: Endorphin production is triggered by various factors, such as physical pain, stress, exercise, excitement, laughter, and certain foods. Each of these triggers stimulates the body to release endorphins as a response.
2. Receptors: The body has specific receptors called opioid receptors, which are located in the brain, spinal cord, and other parts of the nervous system. When these receptors are activated, they help regulate pain and mood.
3. Natural Production: Endorphins are produced naturally in the body by specialized cells, including neurons in the brain and some cells in the adrenal glands. These cells synthesize and release endorphins in response to the triggers mentioned earlier.
4. Chemical Process: Endorphins are peptides, which are short chains of amino acids. The production of endorphins involves the following chemical process:
a. Triggered by a stimulus, the body releases pro-opiomelanocortin (POMC), a precursor molecule.
b. POMC is then cleaved into smaller molecules, including beta-endorphin, in response to the stimulus.
c. Beta-endorphin binds to opioid receptors, particularly the mu-opioid receptors, in the nervous system.
d. When endorphins bind to these receptors, they reduce the perception of pain and produce feelings of pleasure, euphoria, or a general sense of well-being.
It's important to note that endorphins are just one type of neurotransmitter involved in pain regulation and mood modulation.
Other neurotransmitters, such as serotonin and dopamine, also play significant roles in these processes.
Regular physical exercise is a common way to stimulate the release of endorphins, which is why it is often referred to as a "natural high." Additionally, laughter, stress relief techniques, meditation, and even certain foods (like spicy foods) can also trigger endorphin release to varying degrees. The exact mechanisms and pathways involved in endorphin production and regulation are complex and are an active area of scientific research.
What is the chemical relationship between endorphins and seratonin?
Endorphins and serotonin are both neurotransmitters in the brain, but they have different chemical structures and functions.
1. Endorphins:
· Endorphins are a group of peptides (small proteins) produced by the body, primarily in response to stress and pain.
· They are produced in various parts of the brain and the pituitary gland in response to stress, exercise, pain, and other stimuli.
· Endorphins are released during physical activity, such as exercise or intense physical exertion, and can produce feelings of euphoria and pain relief. They are often referred to as "feel-good" chemicals.
· The primary function of endorphins is to act as natural painkillers or analgesics by binding to specific receptors in the brain called opioid receptors.
2. Serotonin:
· Serotonin is a monoamine neurotransmitter derived from the amino acid tryptophan.
· It is synthesized in the brain and found primarily in the central nervous system, though it is also present in other parts of the body, including the gastrointestinal tract.
· Serotonin plays a key role in regulating mood, emotions, sleep, appetite, and other physiological processes.
· It is often associated with feelings of well-being and happiness. Low levels of serotonin have been linked to mood disorders such as depression and anxiety.
· Serotonin functions by transmitting signals between nerve cells and is involved in various neural pathways that regulate mood and behavior. It primarily exerts its effects by binding to specific serotonin receptors.
While both endorphins and serotonin can contribute to feelings of well-being and happiness, they have different chemical structures and mechanisms of action. Endorphins are peptides that primarily function as natural painkillers, while serotonin is a monoamine neurotransmitter with a broader range of functions related to mood and behavior regulation.
HOW DO ENDORPHINS AND DOPAMINE INTERACT IN THE HUMAN BODY
Endorphins and dopamine are two distinct neurotransmitters that play important roles in the human body, particularly in the brain's reward and pleasure pathways. While they have different functions, they can interact and influence each other in certain situations. Here's an overview of how endorphins and dopamine interact:
1. Endorphins:
· Endorphins are a group of naturally occurring peptides (small proteins) produced by the body, primarily in response to stress and pain.
· Their main function is to act as natural painkillers or analgesics, helping to reduce the perception of pain and promote a sense of well-being.
· Endorphins are released during activities such as exercise, stress, laughter, and even certain foods. This release can lead to feelings of euphoria and a "runner's high."
2. Dopamine:
· Dopamine is another neurotransmitter that plays a key role in the brain's reward system. It is involved in motivation, pleasure, reinforcement learning, and the regulation of mood.
· Dopamine is released when you engage in pleasurable activities, such as eating tasty food, having sex, or achieving a goal. It reinforces the behavior that leads to these pleasurable experiences.
· Many addictive substances and activities, such as drugs, alcohol, gambling, and certain types of social media use, can stimulate the release of dopamine, which can contribute to addiction.
Interaction between Endorphins and Dopamine:
Endorphins can indirectly influence dopamine release. For example, engaging in activities that trigger the release of endorphins, such as exercise or certain enjoyable experiences, can also lead to an increase in dopamine levels.
The pleasurable sensations produced by endorphins may contribute to the overall feeling of reward and pleasure associated with activities that stimulate dopamine release.
The interaction between endorphins and dopamine can create a reinforcing loop. Engaging in pleasurable activities that trigger dopamine release can motivate individuals to repeat those activities, leading to the release of endorphins and further reinforcing the behavior.
In summary, endorphins and dopamine are both involved in the brain's reward and pleasure pathways, although they serve different primary functions. Endorphins are primarily associated with pain reduction and stress relief, while dopamine is linked to motivation and pleasure. The release of endorphins during pleasurable activities can indirectly influence dopamine levels and contribute to the overall experience of reward and well-being.
ACETYLCHOLINE
CHOLINERGIC NEUROTRANSMITTERS
( also SEE BDNF , NORADRENALIN PAGE )
Acetylcholine: BDNF influences cholinergic neurons, especially in the basal forebrain, which project to the hippocampus and cortex and are involved in cognitive functions such as learning and memory. BDNF can enhance the survival and function of these neurons, indicating a role in cognitive processes and potentially in the pathophysiology of Alzheimer's disease.
2 Noradrenaline (Norepinephrine): BDNF and noradrenergic systems interact, especially in the locus coeruleus, which is involved in arousal, attention, and stress responses. BDNF can influence the survival and function of noradrenergic neurons, and noradrenergic activity can modulate BDNF expression, suggesting a role in stress-related and attentional processes.
Cholinergic neurotransmitters are chemicals used by the nervous system to transmit signals.
The primary cholinergic neurotransmitter is acetylcholine (ACh). It plays a crucial role in many functions of the body and brain, including muscle activation, pain response, and regulation of the endocrine system.In the brain, acetylcholine is involved in various aspects of cognitive function, including attention, learning, memory, and arousal. It affects brain function in several ways:
Since it helps all nerve transmission
Learning and Memory: Acetylcholine is critical in the formation of new memories and the recall of existing ones. In the brain, particularly in areas like the hippocampus, which is pivotal for memory formation, acetylcholine promotes neuroplasticity and helps facilitate the process of learning.Attention and Arousal: Acetylcholine modulates attention and arousal. It helps in sustaining attention on tasks and is involved in the wakefulness and alertness aspects of the sleep-wake cycle.
Cognitive Function: Higher levels of acetylcholine are associated with improved cognitive function. It is essential for various cognitive processes, including decision-making, focus, and problem-solving.
Neuroplasticity: Acetylcholine contributes to neuroplasticity, which is the brain's ability to adapt and reorganize itself by forming new neural connections. This is fundamental to learning new skills and recovering from brain injuries.
Mood Regulation: Although not as directly involved in mood regulation as neurotransmitters like serotonin or dopamine, acetylcholine can influence mood and has been associated with certain mood disorders.
The cholinergic system's dysfunction is linked to several neurological disorders.
For example, a significant reduction in acetylcholine activity is a hallmark of Alzheimer's disease, leading to memory loss and cognitive decline. Treatments for Alzheimer's and other cognitive impairments often involve medications that enhance cholinergic function, either by increasing the availability of acetylcholine or mimicking its action at receptors.
where are they produced and how are they stimulated
The most prominent cholinergic neurotransmitter is acetylcholine (ACh). These neurotransmitters are critical in a wide range of functions in the body and brain, influencing everything from muscle contraction to memory and arousal.
Effects on Brain Function In the brain, acetylcholine plays key roles in:
Attention and Arousal: It is involved in alertness, attention, and the sleep-wake cycle.
Memory and Learning: Acetylcholine is essential for cognitive functions, particularly in the encoding of new memories and learning processes. The hippocampus, an area crucial for memory formation, has a high concentration of cholinergic neurons.
Motor Control: Though more pronounced in the peripheral nervous system where it controls muscle contraction, acetylcholine also influences motor control in the brain.
Production and Stimulation
Acetylcholine is synthesized in the neuron's cytoplasm from choline and acetyl coenzyme A (acetyl-CoA) through the action of the enzyme choline acetyltransferase. Once synthesized, it is stored in vesicles at the nerve terminal ---Basal Forebrain: Cholinergic neurons in the basal forebrain are critical for cognitive functions and project to the hippocampus and cerebral cortex. --- Brainstem: Neurons in the pedunculopontine and laterodorsal tegmental areas contribute to arousal, sleep-wake cycles, and certain motor functions.
Stimulation
The release and action of acetylcholine IS stimulated by:
Neuronal Activity: When a cholinergic neuron is activated, it leads to the release of acetylcholine into the synaptic cleft.
Drugs and Chemicals: Certain substances can enhance or mimic the action of acetylcholine at its receptors. For example, nicotine from tobacco acts as an agonist at nicotinic acetylcholine receptors, stimulating them directly.
Electrical and Chemical Signals: The activity of cholinergic neurons can also be modulated by various neurotransmitters and neuromodulators that affect their excitability.
After acetylcholine is released into the synaptic cleft, it binds to and activates its receptors on the post-synaptic neuron. There are two main types of acetylcholine receptors: muscarinic and nicotinic, each with different functions and distributions in the brain and body.
The action of acetylcholine is terminated primarily by the enzyme acetylcholinesterase, which breaks down acetylcholine into choline and acetate, allowing choline to be taken back up by the neuron and reused.
GHRELIN
GHRELIN
Hunger hormone impacts decision-making brain area to drive behavior
A hunger hormone produced in the gut can directly impact a decision-making part of the brain in order to drive an animal's behavior, finds a new study by UCL (University College London) researchers.
The study in mice, published in Neuron, is the first to show how hunger hormones can directly impact activity of the brain's hippocampus when an animal is considering food.
Lead author Dr. Andrew MacAskill (UCL Neuroscience, Physiology & Pharmacology) said, "We all know our decisions can be deeply influenced by our hunger, as food has a different meaning depending on whether we are hungry or full. Just think of how much you might buy when grocery shopping on an empty stomach. But what may seem like a simple concept is actually very complicated in reality; it requires the ability to use what's called 'contextual learning."
"We found that a part of the brain that is crucial for decision-making is surprisingly sensitive to the levels of hunger hormones produced in our gut, which we believe is helping our brains to contextualize our eating choices."
For the study, the researchers put mice in an arena that had some food, and looked at how the mice acted when they were hungry or full, while imaging their brains in real time to investigate neural activity. All of the mice spent time investigating the food, but only the hungry animals would then begin eating.
The researchers were focusing on brain activity in the ventral hippocampus (the underside of the hippocampus), a decision-making part of the brain which is understood to help us form and use memories to guide our behavior.
Hunger hormone' enhances memory
by Society for the Study of Ingestive Behavior
A team of neuroscience researchers at the University of Southern California have identified a surprising new role for the "hunger hormone" ghrelin. Ghrelin has previously been recognized for its unique role in sending hunger signals from the gut to the brain, but, as presented this week at the annual meeting of the Society for the Study of Ingestive Behavior, these new findings suggest that it may also be important for memory control.
GHRELIN IS PRODUCED IN THE STOMACH AND SECRETED IN ANTICIPATION OF EATING,
and is known for its role to increase hunger. "For example, ghrelin levels would be high if you were at a restaurant, looking forward to a delicious dinner that was going to be served shortly," said Dr. Elizabeth Davis, lead author on the study.
Once it is secreted, GHRELIN BINDS TO SPECIALIZED RECEPTORS ON THE VAGUS NERVE—a nerve that communicates a variety of signals from the gut to the brain. "We recently discovered that in addition to influencing the amount of food consumed during a meal, the vagus nerve also influences memory function," said Dr. Scott Kanoski, senior author of the study. The team hypothesized that ghrelin is a key molecule that helps the vagus nerve promote memory.
Using an approach called RNA interference to reduce the amount of ghrelin receptor, the researchers blocked ghrelin signaling in the vagus nerve of laboratory rats. When given a series of memory tasks, animals with reduced vagal ghrelin signaling were impaired in a test of episodic memory, a type of memory that involves remembering what, when, and where something occurred, such as recalling your first day of school. For the rats, this required remembering a specific object in a specific location.
The team also investigated whether vagal ghrelin signaling influences feeding behavior. They found that when the vagus nerve could not receive the ghrelin signal, the animals ate more frequently, yet consumed smaller amounts at each meal. Dr. Davis thinks these results may be related to the episodic memory problems. "Deciding to eat or not to eat is influenced by the memory of the previous meal," says Davis. "Ghrelin signaling to the vagus nerve may be a shared molecular link between remembering a past meal and the hunger signals that are generated in anticipation of the next meal."
These novel findings add to our understanding of how episodic memories are generated, as well as the relationship between memory and eating behavior. In the future, researchers may be able to develop strategies for improving memory capacity in humans by manipulating ghrelin signaling from the gut to the brain.
Vagal afferent Ghrelin signaling
The scientists found that activity in a subset of brain cells in the ventral hippocampus increased when animals approached food, and this activity inhibited the animal from eating.
But if the mouse was hungry, there was less neural activity in this area, so the hippocampus no longer stopped the animal from eating. The researchers found this corresponded to high levels of the hunger hormone ghrelin circulating in the blood.
Adding further clarity, the UCL researchers were able to experimentally make mice behave as if they were full, by activating these ventral hippocampal neurons, leading animals to stop eating even if they were hungry. The scientists achieved this result again by removing the receptors for the hunger hormone ghrelin from these neurons.
Prior studies have shown that the hippocampus of animals, including non-human primates, has receptors for ghrelin, but there was scant evidence for how these receptors work.
This finding has demonstrated how ghrelin receptors in the brain are put to use, showing the hunger hormone can cross the blood-brain barrier (which strictly restricts many substances in the blood from reaching the brain) and directly impact the brain to drive activity, controlling a circuit in the brain that is likely to be the same or similar in humans.
Dr. MacAskill added, "It appears that the hippocampus puts the brakes on an animal's instinct to eat when it encounters food, to ensure that the animal does not overeat—but if the animal is indeed hungry, hormones will direct the brain to switch off the brakes, so the animal goes ahead and begins eating."
The scientists are continuing their research by investigating whether hunger can impact learning or memory, by seeing if mice perform non-food-specific tasks differently depending on how hungry they are. They say additional research might also shed light on whether there are similar mechanisms at play for stress or thirst.
The researchers hope their findings could contribute to research into the mechanisms of eating disorders, to see if ghrelin receptors in the hippocampus might be implicated, as well as with other links between diet and other health outcomes such as risk of mental illnesses.
First author Dr. Ryan Wee (UCL Neuroscience, Physiology & Pharmacology) said, "Being able to make decisions based on how hungry we are is very important. If this goes wrong it can lead to serious health problems. We hope that by improving our understanding of how this works in the brain, we might be able to aid in the prevention and treatment of eating disor
ADDITIONAL
Ghrelin or lenomorelin, INN) is a hormone primarily produced by enteroendocrine cells of the gastrointestinal tract, especially the stomach, ] and is often called a "hunger hormone" because it increases the drive to eat. Blood levels of ghrelin are highest before meals when hungry, returning to lower levels after mealtimes. ghrelin may help prepare for food intake [8] by increasing gastric motility and stimulating the secretion of gastric acid.
Ghrelin activates cells in the anterior pituitary gland and hypothalamic arcuate nucleus,[6][9] including neuropeptide Y neurons that initiate appetite. Ghrelin stimulates brain structures having a specific receptor – the growth hormone secretagogue receptor 1A (GHSR-1A). Ghrelin also participates in regulation of reward cognition, learning and memory, the sleep-wake cycle, taste sensation, reward behavior, and glucose metabolism
Ghrelin is a participant in regulating the complex process of energy homeostasis which adjusts both energy input – by adjusting hunger signals – and energy output – by adjusting the proportion of energy going to ATP production, fat storage, glycogen storage, and short-term heat loss. The net result of these processes is reflected in body weight, and is under continuous monitoring and adjustment based on metabolic signals and needs. At any given moment in time, it may be in equilibrium or disequilibrium. Gastric-brain communication is an essential part of energy homeostasis, and several communication pathways are probable, including the gastric intracellular mTOR/S6K1 pathway mediating the interaction among ghrelin, nesfatin and endocannabinoid gastric systems, ] and both afferent and efferent vagal signals.
Ghrelin and synthetic ghrelin mimetics (growth hormone secretagogues) increase body weight and fat mass by triggering receptors in the arcuate nucleus ] that include neuropeptide Y (NPY) and agouti-related protein (AgRP) neurons. Ghrelin-responsiveness of these neurons is both leptin- and insulin-sensitive. Ghrelin reduces the sensitivity of gastric vagal afferents, so they are less sensitive to gastric distension.
In addition to its function in energy homeostasis, ghrelin also activates the cholinergic–dopaminergic reward link in inputs to the ventral tegmental area and in the mesolimbic pathway, a circuit that communicates the hedonic and reinforcing aspects of natural rewards, such as food and addictive drugs such as ethanol. Ghrelin receptors are located on neurons in this circuit. Hypothalamic ghrelin signalling is required for reward from alcohol[ and palatable/rewarding foods.